Introduction
When sulfur is burned or ignited, it generates toxic gases that can suffocate small, land animals such as rodents, and kills other monocellular organisms, e.g. fungi, on contact [
1,
2]. Studies suggest that sulfur may interact with plants and/or fungi to produce toxic agents [
3]. The main theory is that sulfur enters the exposed cells and adversely affects their respiratory chain [
4]. Habitats in the ponds are affected by several factors, such as organic, volumetric loading, physical, chemical, and toxic elements. The best studied examples are sulfur-oxygen gradient and sulfur-nitrate counter gradient systems. Other described examples are microorganisms, specialized in using the energy released by the redox reactions [
5]. Also, physiological studies on species, e.g. Thiobacillus spp. have been conducted by culturing them in homogeneous media [
6].
Successful facultative pond operation relies on maintaining an abundance of stable algae populations, and preserving effluent biochemical oxygen demand (BOD) concentrations [
7]. Effective and ample sulfur transformation occurs via microbial activities in anaerobic ponds. Oxidized sulfur (S8) can be reduced to other elemental species, such as sulfur So and S=, and hydrogen sulfide, biologically. Although sulfur is a part of nutritional substrates, it can cause serious trouble within the ponds ecosystem [
8]. The main problems caused by a high rate of sulfur production in anaerobic ponds are the odour released and its effects on the activity of methane fermenting bacteria. In addition, another problem caused by excessive concentration of sulfur in the ponds is its toxicity [
9]. Changes in sulfur compounds are caused by basic oxidation-reduction mechanism. Organic loading rate under anoxic conditions is considered excessive for a facultative pond but is lower than that for an anaerobic pond, which is environmentally suitable for sulfur bacterial activities [
10]. As a result of low pH in anaerobic stabilization ponds, elemental sulfur shifts to hydrogen sulfur and remains there for longer periods.
However, in under loaded anaerobic or overloaded facultative stabilization ponds, the pH is significantly more alkaline than that of regular ponds. The alkaline pH causes hydrogen sulfide inhibition; therefore, sulfur does not occur in the form of hydrogen sulfide and thus, the odorous emission of H2S is not an issue [
11]. On the other hand, the proliferation of phototrophic blue-green algae, facultative non-sulfur and photosynthetic sulfur bacteria in eutrophic environments can bring about sulfur and hydrogen sulfide oxidation [
12]. Based on current literature reviews, this concept has not been adequately studied.
This study investigated changes in sulfate, sulfide and elemental sulfur concentrations in the wastewater of anaerobic stabilization ponds of oil refineries. Our results revealed that sulfur bacteria, i.e. phototrophs, can significantly reduce the concentrations of sulfur and odorous hydrogen sulfide in public ponds through photosynthetic process.
Materials and Methods
Anoxic stabilization ponds: The study was carried out in two laboratory scale anoxic stabilisation ponds (ASP) at a dimension of 1m long by 0.25 m wide by 1 m deep, and a maximal capacity of 0.25 m3 for a retention time of 6 days. Also, we used an ASP lab scale installed 75 cm deep into the ponds to examine the effect of depth. The ponds were during
12 hours of dark and light cycles. For the light cycle, six halogen lamps (20 watts; Philips, the Netherlands) were placed 30 cm above the ponds’ surface. The industrial wastewater for this study was provided by Kermanshah Petrochemical Wastewater Treatment Plants (Kermanshah, Iran).
Study design: This study had a total design of 20 experiments as follows: a) three volumetric organic loading of 30, 65 and 10 g BOD5/m3/d,b) three sulphate concentrations of 75, 300 and 600 mg/L, at 100C and 25). Lastly, two experiments were performed with low organic loadings of 15 g and 25 g BOD5/m3/d, at a mean concentration of sulfate at 55±5 mg/L. The actual operational parameters are presented in
Table 1.
.jpg)
Analytical methods: The measurable study parameters included: In-pond dissolved oxygen (DO) and the temperature were determined by a Y54 Oxygen Meter with specific DO probe and thermometer. The pH and oxidation-reduction potential (ORP) were measured by a Kent pH meter (WTW InoLab, Model 7110; Germany). Determinations of sulfate and sulfur were made based on the previously published methods [
13], and algal biomass in terms of chlorophyll-a was assessed according to the method explained by Zavřel, et al [
14]. Algal cells and purple sulfur bacteria were quantified based on another previous study [
15]. Bacterio-chlorophyll-a was measured by the detection and estimation of photosynthetic sulfur bacteria, using the method of Kosumi, et al [
16]. Sulphate-reducing bacteria were quantified by the recommended medium of Korenblum [
17].
Results
Relationship between Total sulfur and Independent Variables: Initially, there was a significant effect of volumetric organic loading and influent sulfate concentration versus sulfur (S=, HS-, H2S) generation. However, the effect of each parameter, if studied separately, was relatively weak. Secondly, the effect of sulfur concentrations was strong over the range of influent sulfate concentration, from 100 mg/L to 325 mg/L. Thirdly, the sulfur generation relied on temperature, because it had a strong effect on the biological activity of the sulfate reducing bacteria. Reportedly, the rate of sulfur generation increases by 7% when the temperature rises up to 30oC at anaerobic conditions [
18]. This is equal to approximately doubling the reaction rate for every 10oC increase in temperature.
This study demonstrated that the sulfur concentration increased with every rise in the anoxic ponds’ temperature. The sulfur concentration in the ponds rose with an increase in the volumetric organic loading, from 65 and 100 g BOD5/m3 per day at the influent sulfate concentrations of 100, 325 and 550 mg/L. There was no significant difference in the sulfur concentration build-up throughout the study under cool conditions.
Data in
Figures 1 and 2 demonstrates that the sulfur concentration in the anoxic pond rose sharply from 14 to 26.67 mg/L between the volumetric organic loadings of 30 g BOD/m3 per day, and 65 g BOD/m3 per day at the influent sulphate concentration of 100 mg/L under warm conditions.
.jpg)
.jpg)
The sulfur concentration did not change significantly at a volumetric organic loading of 100 g BOD5/m3 per day under the same temperature. However, the sulfur concentration in the pond increased with a rise in the influent sulphate concentrations, from 100 mg/L to 325 mg/L. The sulfur build-up occurred at both operating temperatures. A simple regression analysis of the data means demonstrated that there was a weak correlation (r=0.47) between sulfur production and sulfate concentration. Consistent with the findings of an earlier study [
19], if sulfate was in excess, the rate of sulfur generation remained relatively independent of the sulfate concentration.
The sulfur accumulation in the ponds rose particularly at dawn, with volumetric organic loading of around 65 g BOD/m3 per day (650 kg BOD/hectare/day), at an influent sulfate concentration of 300 mg/L. In the presence of light; however, the sulfur accumulation decreased by photosynthetic bacteria and, consequently, the noxious odor release was negligible when the light was shining.
Role of photosynthetic sulfur bacteria: The photosynthetic sulfur bacteria proliferated in the ponds that contained the volumetric organic loading of more than 30 g BOD/m3 per day and less than 100 g BOD/m3 per day. The data indicated that the independent operational parameters created suitable conditions for the growth of the microorganisms. The mean number of counted sulfur bacteria in the stabilization ponds was 3.4×16 cells/mL with the volumetric organic loading of 30 g BOD5/m3 per day at the influent sulfate concentration of 325 mg/L under warm conditions. The data indicated that the maximum sulfur and hydrogen sulfide generations occurred during the night and dawn. Of note, hydrogen sulfide concentration increased with a rise in sulfur accumulation, which account for its corrosive and odorous effects.
The adverse effects of hydrogen sulfide; however, may be minimized significantly, using photosynthetic sulfur bacteria in the presence of light in the anoxic pond. However, this beneficial effect may not occur under anaerobic condition. Our findings have revealed that the concentrations of sulfur and hydrogen sulfide increase with a rise in organic loading in the absence of light illumination. This would be a great benefit for the use of anoxic ponds versus the anaerobic ones. Anaerobic ponds are known to generate noxious odour and cause corrosion due to the continual release of hydrogen sulfide. Comparing the effluent sulfur concentrations generated during the dark period with that of three hours after the light exposure clearly indicates that photosynthetic sulfur bacteria are able to play a significant role in sulfur removal.
Table 2 reflects the values of sulfur and hydrogen sulfide toward the effluent in the anoxic ponds with and without the light exposure.
.jpg)
The findings of this research during the 24-hour dark versus light illumination at the anoxic ponds provide valuable evidence for the beneficial activity of photosynthetic sulfur bacteria in the removal of sulfur residues and the noxious effects.
The role of phototrophic sulfur bacteria provides a good reason why the ponds which are located between facultative and fully anaerobic sites are preferred over anaerobic ones, mainly due to their noxious odor. This study revealed that a thick layer of scum developed over the surface of the ponds, which resulted in a reduction in the population of phototroph microorganisms. Specifically, this occurred at a volumetric organic loading of 100 g BOD/m3 per day at the influent sulphate concentrations between 300 to 600 mg/L. Consequently, under these conditions, the depletion of sulfur did not occur significantly by its oxidation secondary to photosynthesis.
Biological species in the ponds: A reduction in the algal population followed by increases in the sulfate-reducing bacteria and the development of photosynthetic bacteria in wastewater stabilization ponds. This event does not mean that the last habitat, were tolerable, rather it indicates that photosynthetic sulfur bacteria prevent sulfur build-up in the ponds. These microorganisms oxidize sulfur and hydrogen sulfide (H2S), and utilize organic matter in the presence of light. These organisms prevent the formation of hydrogen sulfide on water surface of the facultative ponds during daylight.
Table 3 represents the data for the ecological changes in phototrophs.
.jpg)
This Table shows reduced algal biomass, in terms of chlorophyll-a, and the variation in purple sulfur bacteria, supporting the argument for the presence of photosynthetic sulfur bacteria.
Table 3 also provides the mean values of chlorophyll-a and bacterio-chlorophyll-a in the anoxic ponds for each experimental run. The ecological species in the anoxic stabilization ponds were also examined microscopically. The algae in the anoxic ponds were predominantly Chlamydomonas and Euglena with occasional presence of blue-green algae. Nitzschia, a diatom, was also present abundantly at areas of high sulphate concentrations. Paramoeciums were the predominant protozoa. Further, Vorticella and Cholpoeda were sometimes observed. Although we found various species of sulfur bacteria, Chromatium gracile, Chromatium vinosum and Thiocapsa roseopersicina were the predominant populations. Sulphate reducing bacteria were common, particularly in areas of high organic loadings and influent sulphate concentrations. Facultative phototrophic purple non-sulfur bacteria (Rhodopseudomonas capsulatus) were quantified microscopically. Beggiatoa and colourless sulfur bacteria were usually apparent on the surface of the ponds. Nematodes and insect larvae were also observed on the top layers of the ponds.
Mathematical models for sulfur generation and odor release: The following mathematical models were obtained after the multiple regression and multivariate analyses of the effects of volumetric organic loading, influent sulfate concentration and pond temperature versus the pond sulfur and hydrogen sulfide contents (
Equation 1):
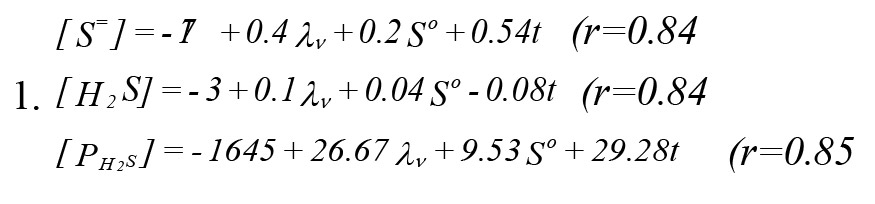
Where:
[S]=Total sulfur concentration inside pond at mid depth early in the morning (mg/L).
λv=Volumetric organic loading in gram of BOD5/m3 per day.
[PH2S]=Partial pressure (ppm) of hydrogen sulfide at equilibrium.
t=Water temperature (°C) in the pond at its mid depth.
So=The combined sulphate and sulfur in the influent (mg/L).
[H2S]=:union:ized hydrogen sulfide in the pond water (mg/L).
r=Multiple regression correlation coefficient.
The above mathematical models for sulfur build-up indicated that its accumulation relied on temperature, volumetric organic loading and the influent inorganic sulfur. The coefficient for each parameter supported the role on sulfur build-up inside the pond. Based on the obtained values, the effect of temperature was more than that of volumetric organic loading (VOL). Also, the effect of VOL was more than that of the influent inorganic sulfur.
Environmental impact of sulfur on ponds equilibrium and ambient air: The main source of odor in stabilization ponds is hydrogen sulfide, which is generated anaerobically. In anoxic ponds, the influent sulfate and sulfur led to the accumulation of sulfur ions. This condition caused the equilibrium with hydrogen sulfide [
20]. In addition, the concentration of hydrogen sulfide at equilibrium is dependent on the water pH. At pH values above 7.5, the majority of the sulfur is in the form of odorless bisulfate. While at pH values below 7.5, the equilibrium rapidly shifts to the formation of non-ionized H2S, its amount is about 80% at pH 7 [
21]. The rotten eggs smell can easily be detected at the level of 1 ppm in the air but not at higher concentrations of H2S. Some algal species can multiply at the sulfur concentrations of 8 to 16 mg/L, whereas others are inhibited at the concentrations of 1-2 mg/L [
22].
Discussion
The results of hydrogen sulfide obtained in this study was based on the measurement of total sulfur, pH and pond temperature. The calculated H2S concentrations are presented in
Table 2. The released hydrogen sulfide varied from 0.07 to 21 mg/L in the anoxic pond contents, while the H2S concentrations varied from 0.05 to 20 mg/L in the effluent, when measured in the morning. There were high concentrations of sulfur and consequently hydrogen sulfur at dawn at low pH values (<7.5). This indicates that odorous emission is a problem in anoxic ponds but this problem may be worse in anaerobic ponds. This is because the accumulated sulfur shifted to the formation of :union:ized hydrogen sulfur due to the low pH [
23].
In this study, although the panel test was not found reliable for odour assessment, the sulfur contents and, consequently, the hydrogen sulfide concentration estimated in the pond were used as an indication of the odor. The environmental problems, such as odor, and the irritation of eyes, nose and throat experienced by people cannot be ignored. These are commonly those who work at a short distance from the ponds where the volumetric organic loadings and influent sulfate are delivered at high concentrations. Experiments at the volumetric organic loadings of 30 and 65g BOD5/m3 per day produced low odor at the end of dark period (dawn), as observed by the authors frequently.
Odour became a serious, permanent and problematic issue at higher volumetric organic loadings under anaerobic conditions, when the generated sulfur accumulated in the anoxic stabilisation ponds. The release of hydrogen sulfur, particularly early in the morning created much offensive odor. This was confirmed by those who worked in the laboratory near the ponds for a long time and complained about the odour, causing nose blocking, eye irritation, headache and burning sensation in the throat. The investigators were also aware of unpleasant odor mainly at the end of the dark period. Again, this occurred particularly when the high volumetric organic loadings were delivered. However, due to poor or lack of light penetration, the anaerobic conditions are not suitable for the phototrophs to utilize sulfur.
Lastly, anoxic ponds are likely to be preferable to anaerobic ponds, because of having low odorous and corrosive effects, and receiving efficient treatments and maintenance. This may be the case if there is negligible sulfur accumulation at the end of the night and if the odor is not objectionable during the day. In that case, the anoxic ponds may be preferred over anaerobic ponds as a better alternative to treat wastewater loadings at an influent sulphate concentration of less than 500 mg/L.
Conclusions
This study provided evidence that hydrogen sulfur is problematic in the wastewater of organic stabilization ponds in the presence of light at dawn, although most of it appeared to be consumed by photosynthetic sulfur bacteria. The biological population of phototrophs changed significantly with increasing volumetric organic loadings. Also, algal genera changed from Euglena to Chlamydomonas with excess loadings and shifted to photosynthetic sulfur bacteria. The species in the high-load facultative and low-load anaerobic, i.e., anoxic ponds also changed from algae to purple sulfur bacteria at increasing influent sulphate concentrations. However, these changes were not as much evident as they were in the case of volumetric organic loadings. The photosynthetic sulfur bacteria were predominantly of Chromatium vinosum and Thiocapsa roseopersicina genera, with some Chromatium okenii observed occasionally. In addition, purple, non-sulfur bacteria (Rhodopseudomnas capsulatus and Rhodopseudomonas spharoid) were present concurrently with photosynthetic sulfur bacteria during the sulfur oxidation. The illumination of bright light on the wastewater in the anoxic stabilization ponds enhanced the chemical reduction of sulfur by the photosynthetic sulfur bacteria.
Ethical Considerations
Compliance with ethical guidelines
All the experimental protocols were in accordance to
the Committee for the Purpose of Control and Supervision of Experiments. Guidelines and objectives and study procedure is approved by ethical committee (KUMS.REC.1395.284).
Funding
Funding for this research was provided by Kermanshah University of Medical Sciences, Kermanshah, Iran (Grant No.: 92066).
Authors' contributions
All authors equally contributed to preparing this article.
Conflict of interest
The authors declared no conflict of interest.
Acknowledgments
We would specially appreciate the Social Development and Health Promotion Research Center of Kermanshah University of Medical Sciences, for providing the authors with invaluable advice, research space and equipment in the course of conducting this research.
References
- Williams J, Cooper R. The oldest fungicide and newest phytoalexin-a reappraisal of the fungitoxicity of elemental sulphur. Plant Pathology. 2004; 53(3):263-79. [DOI:10.1111/j.0032-0862.2004.01010.x]
- DeCant J, Barrett M. Environmental fate and ecological risk assessment for the registration of clothianidin for use as a seed treatment on mustard seed (oilseed and condiment) and cotton. Washington: United States Environmental Protection Agency, Office of Pesticide Programs, Environmental Fate and Effects Division, Enviriomental Risks Branch V; 2010. [Link]
- Tomlin CD. The pesticide manual: A world compendium. Hampshire: British Crop Production Council; 1997. [Link]
- McCallan SEA. The nature of the fungicidal action of copper and sulfur. The Botanical Review. 1949; 15(9):629-43. [DOI:10.1007/BF02861716]
- Almasi A, Dargahi A, Ahagh M, Janjani H, Mohammadi M, Tabandeh L. Efficiency of a constructed wetland in controlling organic pollutants, nitrogen, and heavy metals from sewage. Journal of Chemical and Pharmaceutical Sciences. 2016; 9(4):2924-8. [Link]
- Almasi A, Soleimani H, Mohammadi M, Hossaini H, Falahati MH. Evaluation of anaerobic stabilization pond for removal of pentachlorophenol from wastewater: Response surface methodology. Desalination and Water Treatment. 2018; 129:62-8. [DOI:10.5004/dwt.2018.22886]
- Ho LT, Van Echelpoel W, Goethals PL. Design of waste stabilization pond systems: A review. Water Research. 2017; 123:236-48. [DOI:10.1016/j.watres.2017.06.071] [PMID]
- Martínez-Santos M, Lanzen A, Undacalvo J, Martiv I, Garbisu C, Ruiz-romera E. Treated and untreated wastewater effluents alter river sediment bacterial communities involved in nitrogen and sulphur cycling. Science of the Total Environment. 2018; 633:1051-61. [DOI:10.1016/j.scitotenv.2018.03.229] [PMID]
- Do TL, Do TTU, Le TNC, Hoang PH, Cing TNM. Optimization production conditions of photosynthetic purple bacteria biomass at pilot scale to remove sulphide from aquaculture pond. Journal of Vietnamese Environment. 2018; 9(2):112-7. [DOI:10.13141/jve.vol9.no2.pp112-117]
- Harerimana C, Keffala C, Jupsin H, Vasel JL. Development of a simple model for anaerobic digestion based on preliminary measurements of the bacterial sulphur activity in wastewater stabilization ponds. Environmental Technology. 2013; 34(15):2213-20. [DOI:10.1080/09593330.2012.725773] [PMID]
- Zhao C, Gupta VV, Degryse F, Mclauchlin MJ. Effects of pH and ionic strength on elemental sulphur oxidation in soil. Biology and Fertility of Soils. 2017; 53:247-56. [DOI:10.1007/s00374-016-1170-0]
- Pjevac P, Korlevic M, Berg JS, Buranakic E, Ciglenecki I, Amann R, Orlic S. Community shift from phototrophic to chemotrophic sulfide oxidation following anoxic holomixis in a stratified seawater lake. Applied and Environmental Microbiology. 2015; 81(1):298-308. [DOI:10.1128/AEM.02435-14] [PMID] [PMCID]
- Rice EW, Baired RB, Eaton AD. Standard methods for the examination of water and wastewater. Washington; American Public Health Association, American Water Works Association, Water Environment Federation; 2012. [Link]
- Zavřel T, Sinetova MA, ČervenýJ. Measurement of chlorophyll a and carotenoids concentration in cyanobacteria. Bio-protocol. 2015; 5(9):1467. [DOI:10.21769/BioProtoc.1467]
- Hubas C, Jesus B, Ruivo M, Meziane T, Thiney N, Davoult D, et al. Proliferation of purple sulphur bacteria at the sediment surface affects intertidal mat diversity and functionality. Plos One. 2013; 8(12):82329. [DOI:10.1371/journal.pone.0082329] [PMID] [PMCID]
- Kosumi D, Maruta S, Fujii R, Kanemoto K, Sugisaki M, Hashimoto H. Ultrafast excited state dynamics of monomeric bacteriochlorophyll a. Physica Status Solidi (C) – Current Topics in Solid State Physics. 2011; 8(1):92-5. [DOI:10.1002/pssc.201000684]
- Korenblum E, Devasconcelos Goulart FR, De Almeida Rodrigues I, Abreu F, Lins U, Alves PB, et al. Antimicrobial action and anti-corrosion effect against sulfate reducing bacteria by lemongrass (Cymbopogon citratus) essential oil and its major component, the citral. AMB Express. 2013; 3(1):44. [DOI:10.1186/2191-0855-3-44] [PMID] [PMCID]
- Whaley-Martin K, Jessen G, Nelson TC, Mori J, Apte S, Jarolimek C. et al. The potential role of Halothiobacillus spp. in sulfur oxidation and acid generation in circum-neutral mine tailings reservoirs. Frontiers in Microbiology. 2019; 10:297. [DOI:10.3389/fmicb.2019.00297] [PMID] [PMCID]
- Miao Z, Carroll KC, Brusseau ML. Characterization and quantification of groundwater sulfate sources at a mining site in an arid climate: The monument valley site in Arizona, USA. Journal of Hydrology. 2013; 504:207-15. [DOI:10.1016/j.jhydrol.2013.09.030] [PMID] [PMCID]
- Meyer DD, Deandrade PAM, Durrer A, Andreote FD, Corcao G, Brandelli A. et al. Bacterial communities involved in sulfur transformations in wastewater treatment plants. Applied Microbiology and Biotechnology. 2016; 100(23):10125-35. [DOI:10.1007/s00253-016-7839-3] [PMID]
- Hemme C, van Berk W. Potential risk of h2s generation and release in salt cavern gas storage. Journal of Natural Gas Science and Engineering. 2017; 47:114-23. [DOI:10.1016/j.jngse.2017.09.007]
- Deng YF, Di liao X, Wang Y, Liang JB, Tufarelli V. Prebiotics mitigate in vitro sulfur containing odour generation in caecal content of pigs. Italian Journal of Animal Science. 2015; 14(1):3762. [DOI:10.4081/ijas.2015.3762]
- Gilbert AK, Zhao Y, Otteson CE, Pluth MD. Development of acid-mediated h2s/cos donors that respond to a specific ph window. The Journal of Organic Chemistry. 2019; 84(22):14469-75. [DOI:10.1021/acs.joc.9b01873] [PMID] [PMCID]