Introduction
Ampicillin with a chemical structure of C16H19N3O4S and a molecular weight of 41.349 gr/mol is a β-lactam antibiotic, derived from amino-penicillinic acid. It is widely used in the treatment of numerous infections against beta-lactamase producing bacteria of both Gram-negative and positive species. This antibiotic inhibits transpeptidases by attaching to penicillin-binding protein (PBP) and preventing the formation of bacterial cell wall through interaction with peptidoglycans [1].
Antibiotic resistance particularly against most staphylococcal species, E-coli, hemophilus influenza, moraxella, neisseria gonorrhoeae, salmonella and shigella have been reported [2]. The presence of active pharmaceutical compounds in water resources has been recognized as a major concern in the environmental science [3]. Usually, antibiotics are poorly absorbed or decomposed in the human body, and most of them or their metabolites are excreted through urine and feces, and enter the public sewer systems [3]. If these materials are not removed during conventional wastewater treatment, they will enter public water resources [4]. Also, the antibiotics that are used in livestock and fish hatcheries may reach the water resources [5]. The presence of antibiotics in rivers and lakes [6], groundwater [7], sediments and soils [8] has also been reported. The consumed antibiotics disposed by livestock have made the use of animal fertilizers in agriculture a major challenge [9]. The indirect consumption of antibiotics through water resources and food chains can make humans resistant to them, with the subsequent hazard to the environment [9]. Antibiotics can also contaminate humans through drinking water and/or the consumption of aquatic animals, such as fish with accumulated antibiotic residues in them [9].
Several methods have been used for the removal of Ampicillin from water, such as electrochemical oxidation [9], activated carbon adsorption with H2O2 [10], sugarcane bagasse adsorption [11], membrane bioreactors [12], and submerged aeration [13]. Meanwhile, photo-oxidation is among the advanced methods widely used for the purification of water resources, especially those contaminated with pharmaceutical compounds [14]. Also, successful results have been reported on the application of this method to degrade materials resistant to biological processes [14]. In UV-H2O2 process, the ultraviolet light at 254 nm changes the binding of chemical compounds and accelerates their oxidation [15]. This method is easy to use and has a high oxidation power; therefore, less harmful substances remain in the water, which is considered a desirable method from the environmental perspective [16]. Given the harmful effects of antibiotics on the environment, this study was conducted to investigate the degradation of Ampicillin in aqueous solutions, using UV-H2O2 photo-oxidation process with a focus on four independent variables: a. Ampicillin, b. H2O2 concentrations, c. reaction time, and d. pH level.
Materials & Methods
Materials and Equipment: Ampicillin (C16H19N3O4S and CAS ID: 69-53-4), hydrogen peroxide (CAS ID: 7722-84-1, 30% solution, stabilized), sulfuric acid (CAS ID: 7664-93-9) and caustic soda (CAS ID: 1310-73-2) were purchased from Merck Company (Gernsheim, Germany). Hydrogen peroxide solution was obtained by diluting the stoke sample in a foil coating in a freezer at -10°C. The hydrogen peroxide concentration was determined on a spectrophotometer at 240 nm. All materials were of analytical grades with a minimum purity of 99%. A low-pressure mercury vapor lamp (254 nm) and 8-watt power was obtained from Philips Company (Berlin, Germany).
Degradation Tests: Experiments were performed to investigate the Ampicillin degradation by means of photo oxidation (UV/H2O2) in a 500cc Pyrex reactor equipped with a low-pressure mercury vapor lamp coated with pod quartz at 23±2°C. The lamp was turned on 5 min. before the reactor was switched on to ensure a sustainable level of UV light emission. The reactor was completely covered with aluminum foil to prevent ultraviolet light exposure to the external spaces. A pre-test determined the Ampicillin and hydrogen peroxide concentrations at a range of 5, 15 or 25 mg/l prior to starting the experiments. We set the pH at a range of 3 to 9 to evaluate the acidic or alkaline effect on the process efficiency. The reaction time was chosen 30, 45 or 60 min.
Ampicillin Measurement: The Ampicillin concentration was measured on a spectrophotometer (UV/VIS; UV2100, Unico, USA) at 610 nm [17]. Thus, 5-25 mg/l of Ampicillin was added to 50 ml volumetric flasks. Then, one ml caustic soda (0.5 mol/l) and 2 ml of potassium permanganate at a concentration of 5×10-3 mol/l was added to each flask, diluted with distilled water and kept for 25 min.
Experimental Design: We used the response surface methodology and Central Composite Design (CCD) at three alpha levels of -1, 0, or +1 to evaluate the Ampicillin (A) and H2O2 (B) concentrations, reaction time (C) and pH (D) during the Ampicillin removal. We examined six central and six repetitive runs the center to estimate the test error, nine axis runs and nine factorial runs randomly on software Design Expert, version 6.0, (State-Ease, Inc., Minneapolis, USA) at a 95% confidence level (Table 1). The response was interpreted as the Ampicillin removal efficiency. The experiment results were analyzed statistically, using analysis of variance (ANOVA). The quality of appropriate polynomial model was expressed by correlation coefficient R2, using CCD with the statistical significance examined by the F-Fisher test. Three-dimensional and normal distribution graphs were constructed by Design of Experiment (DOE).
Results
Data Normality: The differences between the obtained numbers from the experiments and the fitted numbers in the model are defined as remainders. The normal distribution of the remainders is shown in Figure 1. The normal data in Figure 1 are aligned on a straight line based on a theoretical observation, preferably with an emphasis on the center-to-end points. The data in this study had a normal distribution.
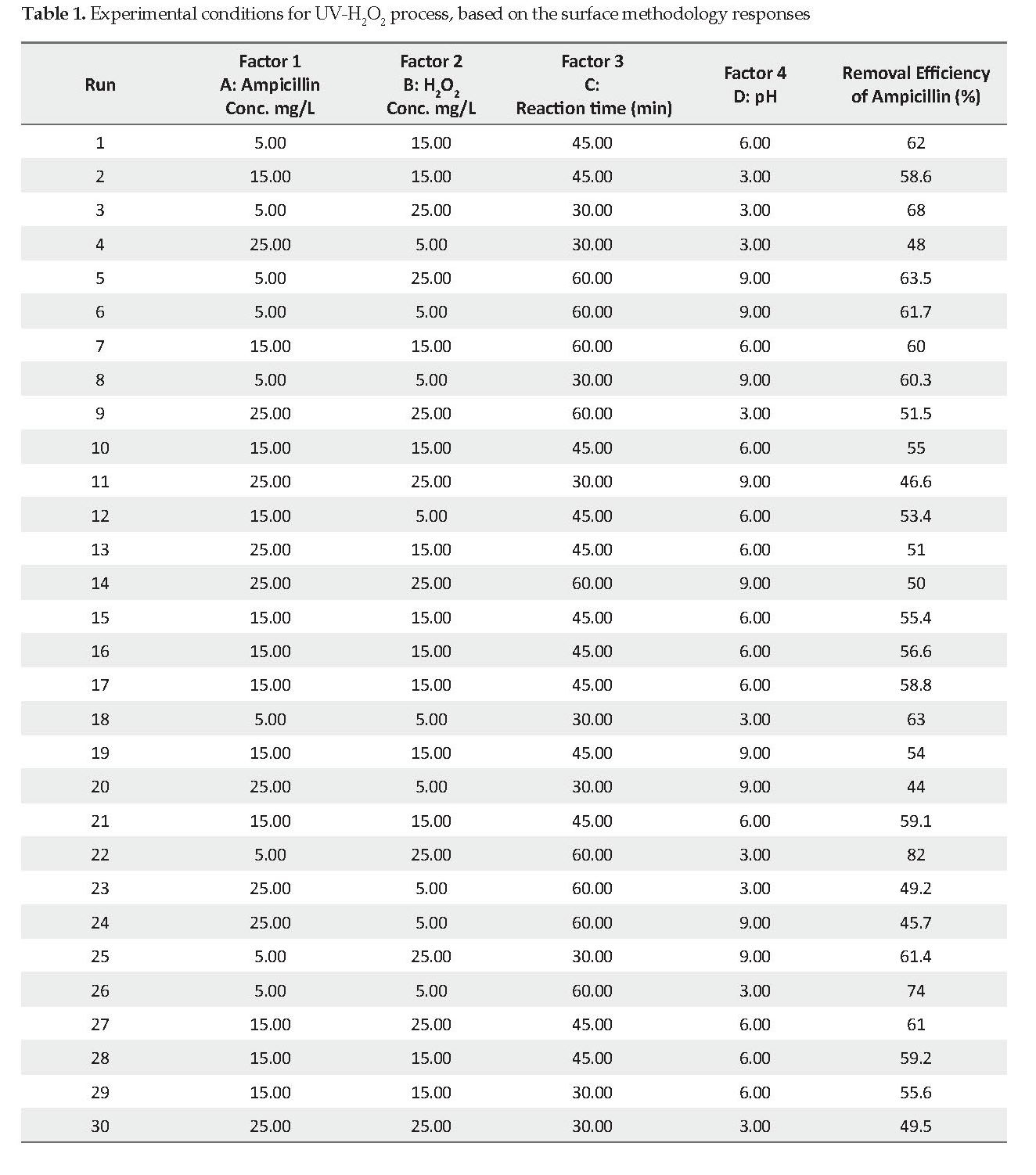
Statistical Analysis: The Ampicillin removal was investigated as a response to the surface methodology processes. The impact of independent variables on the process efficiency is shown in Figure 2. The relationship among variables was coded and the outcome was derived by equation 1 as follows:
1. Y=56.88–8.91A+1.66B+2.29C–3.14D
The efficiency of the Ampicillin removal followed a linear model. The model terms were obtained after the elimination of meaningless variables and their inter-relationship with the response. According to the above equation, factor A had the most and factor B had the least impact on the process efficiency. The positive and negative signs denote a direct or reverse relationship between the examined parameters and the response [18]. The results of ANOVA test and equation regression from the response are summarized in Tables 2 and 3. As shown in these Tables, the model was significant at 99% confidence level with low probability values (0.05 to <0.0001).
The value of R2 was high in this study, which represented the regression significance at 95% level with sufficient agreement among the actual data and those of the models. Also, the probability level which was lower than the F values in the absence of model fit was not significant at 95% confidence level. As shown in Table 2, the model authenticity and accuracy were desirable for being greater than 4 [19]. Figure 3 reflects the interaction among the variables in the process efficiency of UV/H2O2.
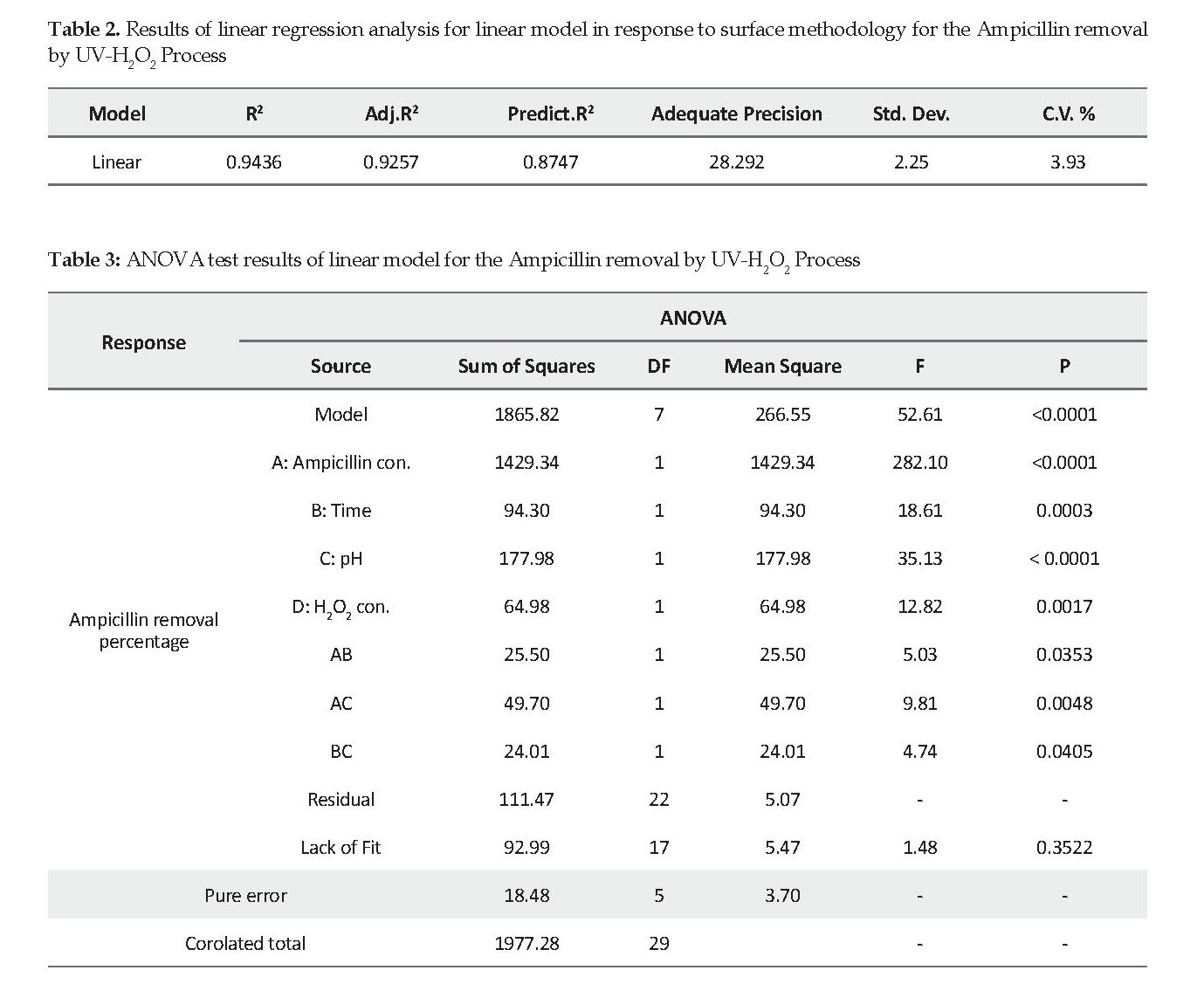
Discussion
In this study, the Ampicillin molecules were exposed to ultraviolet light and hydroxyl radicals of H2O2, such that its removal was made possible ranging from 44% to 82%. The initial concentration of Ampicillin in aqueous solution is very important in photo-oxidation processes. The concentration was selected between 5 and 25 mg/l. By increasing the initial Ampicillin concentration, its removal efficiency was significantly reduced. The highest removal efficiency at the initial concentration of 5 mg/l was 82%. Therefore, this was chosen as the optimal Ampicillin concentration. The lowest efficiency (44%) was obtained at the Ampicillin concentration of 25 mg/l. According to equation 1, the antibiotic concentration with a coefficient of 8.91 was recognized as the most influential parameter on the process efficiency.

As shown in Figures 2 and 3, the negative effect of Ampicillin concentration on efficacy can be justified by the fact that increases in the concentration serve as the filter to reduce the UV light absorption [19]. Therefore, a significant proportion of the pollutant remains unoxidized. Reducing ultraviolet photons absorption by increasing the Ampicillin concentrations can negatively affect the production of hydroxyl groups due to the hydrogen peroxide degradation and reduced oxidation rate, hence the reduced removal efficiency. The UV light and hydrogen peroxide share a synergistic effect [20]. The presence of intermediate products and metabolites could also be involved. It can be argued that by increasing the antibiotic concentration, the possibility of competing metabolites resulted from primary molecule degradation increases [21]. In a study by Zuorro et al. on the chloramphenicol removal by UV-H2O2 using the same surface methodology, similar results were reported at 0-60 min [22]. reaction time. Their results demonstrated that as the antibiotic concentration increased, the removal efficiency declined [22].
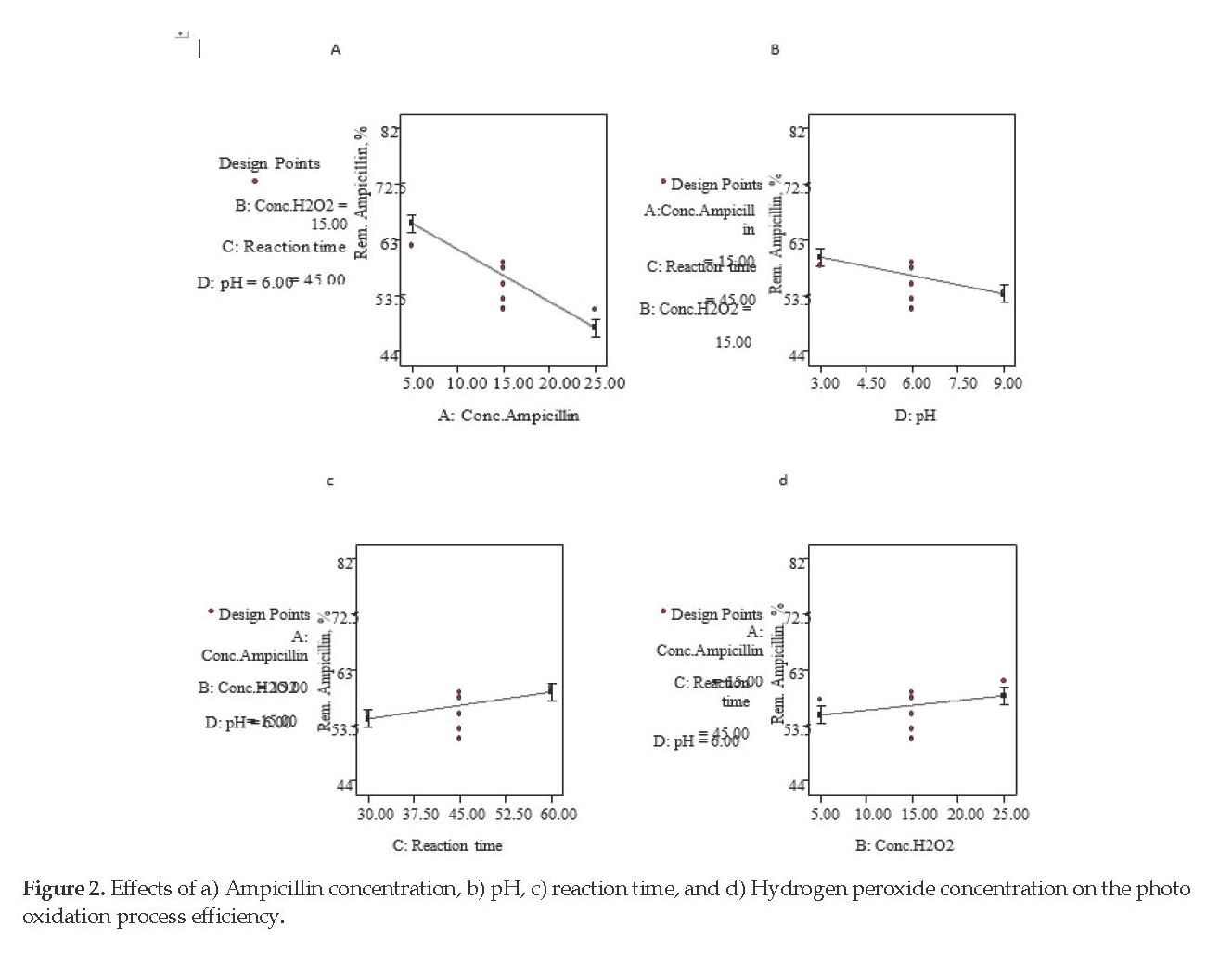
Our results also provided evidence on the effect of pH versus Ampicillin degradation. Based on equation 1, the pH was the most influential parameter on the process efficiency secondary to the Ampicillin concentration. The results showed that pH has a reverse relationship with the efficiency. The maximum efficiency was obtained at pH 3. By gradually increasing the pH up to 9, the efficiency of the Ampicillin removal decreased (Figure 2B). In other words, the Ampicillin removal in the acidic environment was achieved better than that in the neutral or alkaline solution. By keeping other parameters constant (Ampicillin concentration=25 mg/l, contact time=30 min., and hydrogen peroxide concentration=25 mg/l), lowering the pH from 9 to 3, the Ampicillin removal efficiency increased by 48%. This may be attributed to the formation of oxonium ions, which are responsible for the stability and efficacy of hydrogen peroxide.
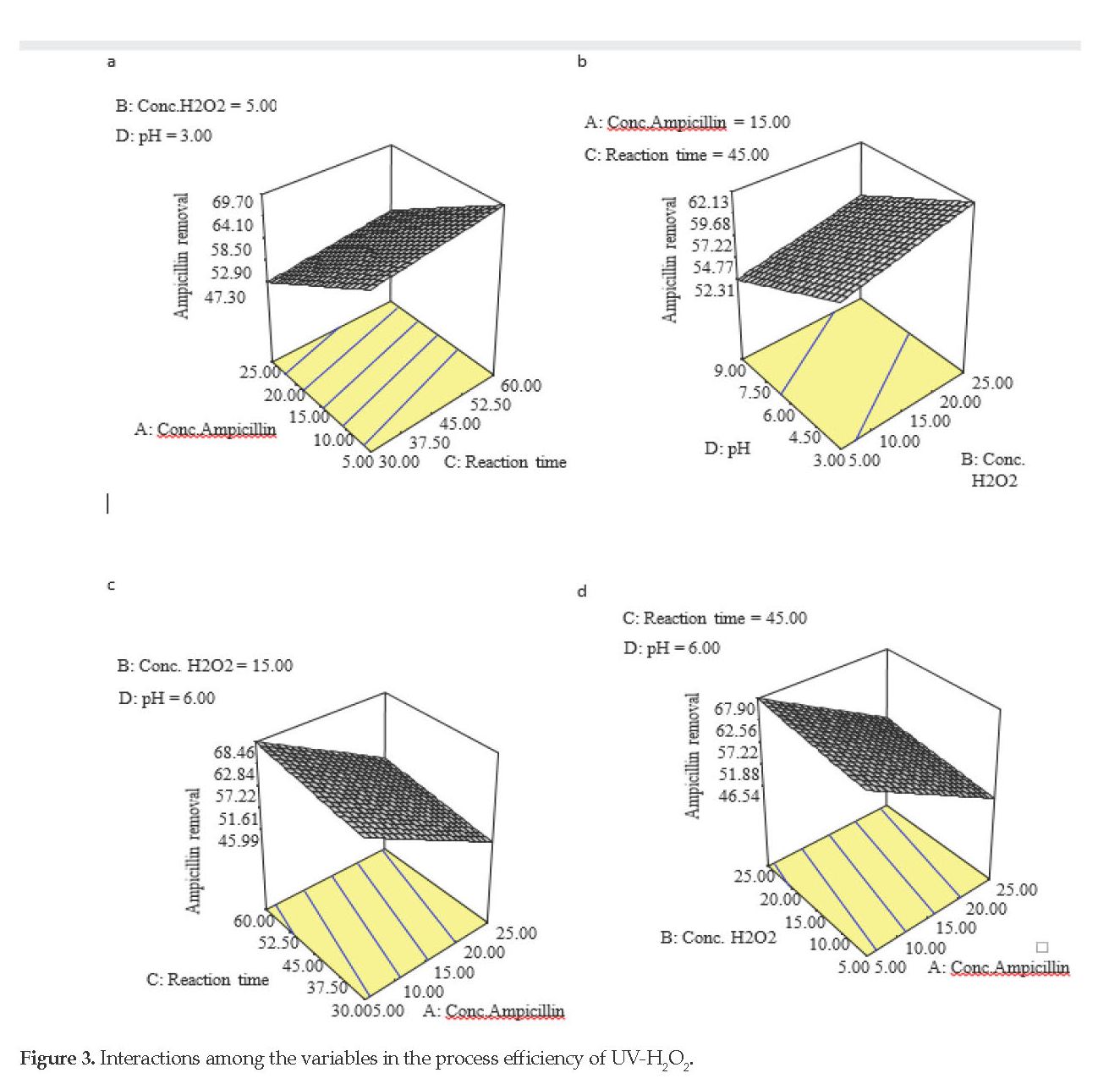
In alkaline pH, hydrogen peroxide turns into water and loses its oxidizing property. In fact, the solution pH affects both the medium chemistry and the molecular bonds. The optimum pH in the current study was found to be 3. In previous studies, the effect of a wide range of pH levels on the efficiency of antibiotics removal has been shown [10, 23].
Based on our results, the 60-minute reaction time was found to be optimal with a maximal removal efficiency of 82%. By reducing the reaction time, the efficiency of photo oxidation process also decreased. For example, at an Ampicillin concentration of 25 mg/l, pH 3 and hydrogen peroxide concentration of 5 mg/l, the system efficiency increased insignificantly from 48 to 49.2% if the reaction time increased from 30 to 60 min. Of note, the reaction time was found to be the third important factor in the current study. The impact of longer reaction time was more significant at higher H2O2 and lower Ampicillin concentrations (Figure 2C). At higher reaction times, the active hydroxyl production increases. The reaction performance improved when ample time for the oxidation process and free radical’s attack on Ampicillin molecules were provided [24].
The hydrogen peroxide concentration was directly related to the system efficiency with a coefficient of 1.66. The H2O2 effect on the Ampicillin removal was less than the effects of other parameters. Its positive effect may be due to increased production of hydroxyl groups at higher concentrations. A previous study has indicated that the hydrogen peroxide concentration is a strategic parameter in oxidation reactions [25]. In that study, the photolysis in the presence of H2O2 accelerated the photo-oxidation process due to the production of hydroxyl groups and prevented the electron-hole formation [25].
The UV radiation through hydrogen peroxide photolysis or optical-inductive processes leads to the production of hydroxyl groups, leading to easier degradation of Ampicillin molecules. Jung et al. [26] achieved similar results in their study on the Amoxicillin removal by UV-H2O2 oxidation. In their study, hydrogen peroxide was used at concentrations of 0-5 mmol/l with the reaction times ranged 0-140 min. Consequently, 99% of the Amoxicillin was removed from the media in 20 min. with the hydrogen peroxide at 10 mmol/l.
Conclusions
We conclude that photochemical oxidation, as used in this study, is a very efficient method for the removal of Ampicillin from aqueous solutions compared to other advanced processes. The combination of ultraviolet light and hydrogen peroxide can achieve this purpose. Such variables as the concentrations of Ampicillin and hydrogen peroxide, the reaction time and pH are important for the optimal efficiency of Ampicillin removal, using the response surface methodology. The Ampicillin concentration (5 mg/l) at pH 3 had negative effects while the hydrogen peroxide concentration (25 mg/l) and with the reaction time at 60 min. had positive effects on the efficiency of the Ampicillin removing process. Based on the response surface methodology, the Ampicillin concentration was the most effective parameter on process efficiency, while hydrogen peroxide concentration was the least important parameter. Therefore, the photo oxidation process, as used in this study, is recommended for the removal of Ampicillin from aqueous solutions.
Ethical Considerations
Compliance with ethical guidelines
All ethical principles were considered in this article. The participants were informed about the purpose of the research and its implementation stages; they were also assured about the confidentiality of their information. Moreover, they were allowed to leave the study whenever they wish, and if desired, the results of the research would be available to them.
Funding
The present paper was extracted from the Ph.D thesis of the first author, Department of Environmental Engineering, Ahvaz Branch, Islamic Azad University, Ahvaz, Iran.
Author's contributions
Conceptualization: Reza Jalilzadeh Yengejeh; Investigation: Rouhollah Shokri; Writing-original draft: Rouhollah Shokri and Reza Jalilzadeh Yengejeh; Writing-review & editing: Ali Akbar Babaei, Ehsan Derikvand and Ali Almasi; Supervisor: Reza Jalilzadeh Yengejeh and Ali Akbar Babaei; Advisor: Ehsan Derikvand and Ali Almasi; Resources and Methodology: All authors.
Conflict of interest
The authors declared no conflict of interests.
References
Archer E, Petrie B, Kasprzyk-Hordern B, Wolfaardt GM. The fate of Pharmaceuticals and Personal Care Products (PPCPs), Endocrine Disrupting Contaminants (EDCs), metabolites and illicit drugs in a WWTW and environmental waters. Chemosphere. 2017; 174:437-46. [DOI:10.1016/j.chemosphere.2017.01.101] [PMID]
Sharma VK, Johnson N, Cizmas L, McDonald TJ, Kim H. A review of the influence of treatment strategies on antibiotic resistant bacteria and antibiotic resistance genes. Chemosphere. 2016; 150:702-14. [DOI:10.1016/j.chemosphere.2015.12.084] [PMID]
Jendrzejewska N, Karwowska E. The influence of antibiotics on wastewater treatment processes and the development of antibiotic-resistant bacteria. Water Sci Technol. 2018; 77(9-10):2320-6. [DOI:10.2166/wst.2018.153] [PMID]
Arslan-Alaton I, Gurses F. Photo-Fenton-like and photo-fenton-like oxidation of Procaine Penicillin G formulation effluent. J Photochem Photobiol A Chem. 2004; 165(1-3):165-75. [DOI:10.1016/j.jphotochem.2004.03.016]
Awad YM, Kim SC, Abd El-Azeem SAM, Kim KH, Kim KR, Kim K, et al. Veterinary antibiotics contamination in water, sediment, and soil near a swine manure composting facility. Environ Earth Sci. 2014; 71(3):1433-40. [DOI:10.1007/s12665-013-2548-z]
Ouyang WY, Huang FY, Zhao Y, Li H, Su JQ. Increased levels of antibiotic resistance in urban stream of Jiulongjiang River, China. Appl Microbiol Biotechnol. 2015; 99(13):5697-707. [DOI:10.1007/s00253-015-6416-5] [PMID]
Ji Y, Ferronato C, Salvador A, Yang X, Chovelon JM. Degradation of ciprofloxacin and sulfamethoxazole by ferrous-activated persulfate: Implications for remediation of groundwater contaminated by antibiotics. Sci Total Environ. 2014; 472:800-8. [DOI:10.1016/j.scitotenv.2013.11.008] [PMID]
Cha J, Yang S, Carlson KH. Occurrence of β-lactam and polyether ionophore antibiotics in surface water, urban wastewater, and sediment. Geosyst Eng. 2015; 18(3):140-50. [DOI:10.1080/12269328.2015.1010658]
Körbahti BK, Taşyürek S. Electrochemical oxidation of ampicillin antibiotic at boron-doped diamond electrodes and process optimization using response surface methodology. Environ Sci Pollut Res Int. 2015; 22(5):3265-78. [DOI:10.1007/s11356-014-3101-7] [PMID]
Wang G, Wu T, Li Y, Sun D, Wang Y, Huang X, et al. Removal of ampicillin sodium in solution using activated carbon adsorption integrated with H2O2 oxidation. J Chem Technol Biotechnol. 2012; 87(5):623-8. [DOI:10.1002/jctb.2754]
Khademi D, Mohammadi MJ, Shokri R, Takdastan A, Mohammadi M, Momenzadeh R, et al. Application of cane bagasse adsorption on nitrate removal from groundwater sources: Adsorption isotherm and reaction kinetics. Desalin Water Treat. 2018; 120:241-7. [DOI:10.5004/dwt.2018.22730]
Labinghisa RS, Rollon AP. Ampicillin removal by Polyvinylidene Difluoride (PVDF), Polyethersulfone (PES) and Nylon for membrane bioreactor application. Int J Innov Manag Technol. 2014; 5(2):105-10. http://www.ijimt.org/papers/495-H0010.pdf
Baghapour MA, Shirdarreh MR, Faramarzian M. Amoxicillin removal from aqueous solutions using submerged biological aerated filter. Desalin Water Treat. 2015; 54(3):790-801. [DOI:10.1080/19443994.2014.888014]
Dewil R, Mantzavinos D, Poulios I, Rodrigo MA. New perspectives for advanced oxidation processes. J Environ Manage. 2017; 195(Pt 2):93-9. [DOI:10.1016/j.jenvman.2017.04.010] [PMID]
Shokri R, Jalilzadeh Yengejeh R, Babaei AA, Derikvand E, Almasi A. UV activation of hydrogen peroxide for removal of azithromycin antibiotic from aqueous solution: Determination of optimum conditions by response surface methodology. Toxin Rev. 2019 February. [DOI:10.1080/15569543.2018.1517803]
Su R, Chai L, Tang C, Li B, Yang Z. Comparison of the degradation of molecule and ionic ibuprofen in UV/H2O2 system. Water Sci Technol. 2018; 77(9-10):2174-83. [DOI:10.2166/wst.2018.129] [PMID]
Khan AAP, Mohd A, Bano Sh, Siddiqi KS, Asiri AM. Spectrophotometric methods for the determination of ampicillin by potassium permanganate and 1-chloro-2, 4-dinitrobenzene in pharmaceutical preparations. Arabian J Chem. 2015; 8(2):255-63. [DOI:10.1016/j.arabjc.2012.04.033]
Almasi A, Mohammadi M, Shamsi Kh, Mohammadi S, Saeidimoghadam Z. Sonolytic and photocatalytic (sonophotocatalytic) removal of cephalexin from aqueous solution: Process optimization using Response Surface Methodology (RSM). Desalin Water Treat. 2017; 85:256-63. [DOI:10.5004/dwt.2017.21030]
Almasi A, Dargahi A, Mohamadi M, Biglari H, Amirian F, Raei M. Removal of Penicillin G by combination of sonolysis and Photocatalytic (sonophotocatalytic) process from aqueous solution: Process optimization using RSM (Response Surface Methodology). Electron Physician. 2016; 8(9):2878-87. [DOI:10.19082/2878] [PMID] [PMCID]
Ferro G, Guarino F, Cicatelli A, Rizzo L. β-lactams resistance gene quantification in an antibiotic resistant Escherichia coli water suspension treated by advanced oxidation with UV/H2O2. J Hazard Mater. 2017; 323(Pt A):426-33. [DOI:10.1016/j.jhazmat.2016.03.014] [PMID]
Özkal CB, Frontistis Z, Antonopoulou M, Konstantinou I, Mantzavinos D, Meriç S. Removal of antibiotics in a parallel-plate thin-film-photocatalytic reactor: Process modeling and evolution of transformation by-products and toxicity. J Environ Sci (China). 2017; 60:114-22. [DOI:10.1016/j.jes.2016.12.025] [PMID]
Zuorro A, Fidaleo M, Fidaleo M, Lavecchia R. Degradation and antibiotic activity reduction of chloramphenicol in aqueous solution by UV/H2O2 process. J Environ Manage. 2014; 133:302-8. [DOI:10.1016/j.jenvman.2013.12.012] [PMID]
Shaykhi ZM, Zinatizadeh AAL. Statistical modeling of photocatalytic degradation of Synthetic Amoxicillin Wastewater (SAW) in an immobilized TiO2 photocatalytic reactor using Response Surface Methodology (RSM). J Taiwan Inst Chem Eng. 2014; 45(4):1717-26. [DOI:10.1016/j.jtice.2013.12.024]
Gleason JM, McKay G, Ishida KP, Mezyk SP. Temperature dependence of hydroxyl radical reactions with chloramine species in aqueous solution. Chemosphere. 2017; 187:123-9. [DOI:10.1016/j.chemosphere.2017.08.053] [PMID] [PMCID]
Gashtasbi F, Jalilzadeh Yengejeh R, Babaei AA. Adsorption of vancomycin antibiotic from aqueous solution using an activated carbon impregnated magnetite composite. Desalin Water Treat. 2017; 88:286-97. [DOI:10.5004/dwt.2017.21455]
Jung YJ, Kim WG, Yoon Y, Kang JW, Hong YM, Kim HW. Removal of amoxicillin by UV and UV/H2O2 processes. Sci Total Environ. 2012; 420:160-7. [DOI:10.1016/j.scitotenv.2011.12.011] [PMID]