Jalili S, Mirzaei F, Ramezani V, Ghoshouni H, Zabihi M. Pure Thymol, and its Nanoliposome and Nanoparticle Forms, Inhibit Trichomonas vaginalis Infection in Culture. IJT 2023; 17 (3) :10-18
URL:
http://ijt.arakmu.ac.ir/article-1-1190-en.html
1- Department of Pharmacology, School of Pharmacy, Shahid Sadoughi University of Medical Sciences, Yazd, Iran
2- Department of Medical Parasitology and Mycology, School of Medicine, Shahid Sadoughi University of Medical Sciences, Yazd, Iran
3- Department of Pharmaceutics, School of Pharmacy, Shahid Sadoughi University of Medical Sciences, Yazd, Iran.
4- Department of Pharmacology, School of Pharmacy, Shahid Sadoughi University of Medical Sciences, Yazd, Iran , mzabihi100@gmail.com
Full-Text [PDF 506 kb]
(437 Downloads)
|
Abstract (HTML) (1389 Views)
Full-Text: (431 Views)
Introduction
Trichomona vaginalis (T. vaginalis) is a flagellate protozoan that causes genitourinary tract infections, mainly in women (1). The incidence of this parasitic infection has been reported to be between 4-6% in different parts of the world (1, 2). This infection is transmitted through sexual contact, shared towels and underwears, or via non-sterile medical devices. Factors such as poverty, illiteracy, multiple sexual partners, and aging increase the risk of this infection (3, 4).
In women, this parasite causes vaginitis, inflammation of bladder, foul-smelling mucus, burning, and itching. Similarly, it causes urinary tract inflammation, burning, and prostate inflammation in men (4, 5). In addition, this parasite can cause many other complications, such as preterm delivery, low birth weight, miscarriage, premature rupture of amniotic sac, ectopic pregnancy, postpartum endometriosis, salpingitis, chronic cervicitis, cervical cancer, and reversible infertility (6, 7).
Metronidazole and its analog tinidazole are two popular drugs for treating trichomoniasis in humans. Among the risks of using these drugs is their carcinogenicity in animals. About 5% of T. vaginalis infections are estimated to be resistant to these two drugs (8, 9). Microbial resistance is one of the main reasons for the failure of treatment with this and other antibiotic drugs (10, 11). This drug is contraindicated in pregnant women, especially in the first trimester (12). Given the adverse effects of Metronidazole, the need for an alternative drug is warranted to treat T. vaginalis infection.
Application of plant-derived formulations in traditional medicine has been popular since they are low-risk, available and inexpensive natural agents, compared to synthetic antibiotics in the treatment of various microbial infections (13, 14). Thymol is a phenolic compound found in plants, such as thyme and its seeds, ajowan (T. ammi). Phenolic compounds, such as thymol and its isomer carvacrol, are believed to have anti-bacterial, anti-fungal, and antioxidant properties. They are used as natural food preservatives, and have anti-aging properties in mammalians (15). Their antimicrobial effect is likely due to increased permeability in the cell membrane, which can disrupt the concentration of cations (15).
Since there are problems with pure plants’ essential oils, such as instability, evaporation, and decomposition under environmental conditions, a modern approach is to prepare nanoparticle compounds (16). While providing excellent preservation, nanoparticles do not hurt the antimicrobial activity of thymol preparations. Further, due to the small size at nanoscale, the nano-preparations can increase the inactive cell uptake, reduce the transfer resistance, and improve the antimicrobial activity (17).
A liposome is a microscopic vesicle consisting of two phospholipid layers surrounding an aqueous component. The lipid bilayer thickness is typically between 3-6 nm, and the resultant liposomes are between 50nm and 50μm in diameter. Due to the amphipathic nature of their constituent elements, liposomes allow for the delivery of hydrophilic or lipophilic drugs. These tiny sac-like structures are like pockets or capsules prepared to carry the drug to different parts of the body. Liposomes have been able to reduce adverse side effects and improve drug stability. They are used as carriers for drugs, enzymes, anticancer, fungicidal, antiparasitic, antiviral, and antibacterial agents (18).
Considering the above facts and reviews, the aim of this study was to investigate the effect of pure thymol and its two nano-preparations on the inhibition of T. vaginalis parasites.
Materials and Methods
Measurement and determination of thymol concentration: Thymol (Merck, Germany) was dissolved in ethanol as the solvent. The UV absorption of seven concentrations of thymol solution were read at 276 nm at a concentration of 1, 5, 10, 20, 30, 40, or 50 mg/ml on a UV/vis spectrophotometer (Unico, China), based on the following equation: Y = 0.0149X + 0.0019.
Preparation of thymol solid lipid nanoparticles: Solid lipid nanoparticles were prepared using the emulsification solvent and evaporation methods. Thymol and various fats were dissolved in acetone according to Table 1, and dispersed in an aqueous medium containing polyvinyl alcohol. Solid lipid nanoparticles were prepared after evaporation of acetone from the aqueous medium.
Table 1. Specifications of the Solid Thymol Lipid Nanoparticles.
*S6 |
*S5 |
*S4 |
*S3 |
*S2 |
*S1 |
Component |
10 mg |
10 mg |
10 mg |
10 mg |
10 mg |
10 mg |
Thymol |
1 ml |
1 ml |
1 ml |
1 ml |
1 ml |
1 ml |
Solvent (vol) |
10 ml |
10 ml |
10 ml |
10 ml |
10 ml |
10 ml |
PV Alcohol |
SA (10) |
Labrafil (10) |
Tefose (10) |
GMS (50) |
GMS (20) |
GMS (10) |
Fat (mg) |
10 ml |
10 ml |
10 ml |
10 ml |
10 ml |
10 ml |
Total (vol) |
100% |
8.72% |
18.55% |
16.84% |
19.32% |
92% |
Thymol (en) |
* Solid thymol lipid nanoparticles.
GMS, Glycerol monostearate; SA, Stearic acid; PV, Polyvinyl; vol, volume; en, enclosure.
Table 2. Formulation of different nanoliposome forms of thymol.
ENC |
Conc. (µg/ml) |
CLF |
Thymol |
CLS |
PS-80 |
PS-20 |
PTC |
Group |
33% |
66.2080 |
1 ml |
10mg |
|
|
|
50 mg |
L1 |
39% |
78.1140 |
1 ml |
20mg |
|
|
|
50 mg |
L2 |
93% |
187.020 |
1 ml |
30mg |
|
|
|
50 mg |
L3 |
1% |
2.4697 |
1 ml |
10mg |
10 mg |
|
|
50 mg |
L4 |
2% |
5.5100 |
1 ml |
10mg |
|
10 mg |
|
50 mg |
L5 |
14% |
29.0671 |
1 ml |
10mg |
|
|
10 mg |
50 mg |
L6 |
PTC, Phosphatidylcholine; PS, Polysorbate; CLS, Cholesterol; CLF, Chloroform; Conc, Concentration; ENC, Encapsulation.
Evaluation of thymol release from particles: To investigate the release of thymol from the nanoparticles, 1 ml of nanoparticles was poured in a dialysis bag (cut off =13 kD) and placed in 100 ml phosphate buffer at pH 7. Then, a 2ml sample was withdrawn at each of the following 14 time points: 1, 2, 5, 10, 15, 20, 30, 45, 60, 120, 180, 240, 300, and 360 min, and a 2 ml phosphate buffer were replaced in the tube. Lastly, the thymol concentration of each sample was read at 276 nm on a UV/vis spectrophotometer.
Preparation of thymol in liposomal form: Thymol nanoliposomes were made by thin layer hydration method as described previously (19). The required amount of phosphatidylcholine, thymol, and the lipid-soluble ingredients were dissolved in chloroform as shown in Table 2. The thin layer was formed by evaporation of the solvent under vacuum in a rotary evaporator at 50 rpm. The lipid layer was hydrated with phosphate buffer. The nanoliposomes were prepared after sonication of the formed large-sized vesicles for 5 minutes at 4°C (Table 2).
Encapsulation efficiency of thymol nanoliposomes: One ml of the prepared nanoliposomes was transferred to the dialysis bag and kept in distilled water at 4°C. Then, they were broken using ethanol, and the amount of encapsulated thymol was measured on a UV spectrophotometer.
Release of thymol from nanoliposomes: To determine the release pattern of thymol from nanoliposomes, 1-ml of each formulation was transferred to the dialysis bag, suspended in 100 ml of phosphate buffer at pH 7, and stirred. Then, 2-ml of the sampled was withdrawn at fixed time points. The sample’s thymol concentration was determined on a UV/vis spectrophotometer at λmax 276.
Particle size measurement: The nanoliposomes’ particle size and solid lipid nanoparticles (SLNs) were measured using a dynamic light scattering method (Zetasizer, NanoZS, Malvern) after dilution with an aqueous medium at 25°C.
Proliferation of T. vaginalis: This parasite strain was isolated from vaginal secretions of a woman with trichomoniasis at Isfahan University of Medical Sciences and stored in a freezer at minus 70°C. The parasites were cultured in the required amount in TYIS-33 medium for mass proliferation.
Exposure of T. vaginalis to the products: Based on previous studies, after the parasite strain reached a concentration of 10000 per ml in the logarithmic phase, it was exposed to the 10 concentrations (0.5-25 µg/ml) of each of the thymol nanoparticle forms, liposomal structures (formulations L3 and L6), and pure thymol. In addition, the 10 samples were infected with drug-free nanoparticle and liposomal forms, plus one sample for each of Metronidazole and controls. The experiment was repeated three times, and the inhibitory effects of the interventions were examined three times on the growth of T. vaginalis within 24, 48, and 72 hours. This experiment consisted of 468 samples in total. All groups were kept at 25°C for 72 hours, and the live T. vaginalis parasites were counted at 24, 48, or 72 hours on a hemocytometer.
The growth inhibition for each of the thymol concentrations, nanoparticle and liposomal forms was calculated in the presence of the parasites, using the following formula: GI = [(a-b) / a]. Where, “GI” was growth inhibition, “a” was the number of the live parasites in the control sample, and “b” was the number of the live parasites in the sample containing a specific thymol product.
Statistical analysis: Using Excel software, version 2019, the inhibitory concentration (IC50) was calculated for thymol nanoparticle and liposomal forms, and pure thymol. The results were presented as the means and standard deviations. The statistical data analyses were performed, using SPSS version 21, one-way ANOVA and Tukey’s post hoc test at a 95% confidence interval.
Results
The anti-Trichomonas activity of the formulations was evaluated on S1, S6, L3 and L6 formulations. The S1 and S6 formulations were tested for entrapment efficiency at 92% (containing stearic acid) and 100% (containing glycerol monostearate), respectively. Also, the L3 and L6 formaulations were evaluated for entrapment efficiency at 93% and 14%, respectively.
As shown in Figure 1, the contamination of glycerol mono-stearate in S1 enhanced the release of thymol from nanoparticles compared to that of the stearic acid. This trend was also noted when Tween 20 was used in the L6 formulation. Adding this substance to nanoliposomes changed the liposomes membrane fluidity and the entrapment of thymol as a lipid-soluble agent. The added fluidity led to a decreased lipid bilayer capacity and entrapment efficiency. Thymol was released more rapidly from the S1 formulation compared to that of S6 (Figure 1). However, the remaining thymol in liposome containing polysorbate 80, with an entrapment efficiency of 14%, showed a kinetic pattern similar to liposome without surfactant (L3). See Figure 2.
Figure 1 compares the thymol release from the nanoparticle form in formulations S1 and S6. Figure 2 compares the concentration of thymol release from the liposomal forms in L3 and L6 preparations. The results on the empty nanoparticle system and nanoliposomes showed that neither group had a lethal effect on T. vaginalis parasites. However, Metronidazole at 65μg/ml completely inhibited the parasites at the three time points of 24, 48, and 72 hours.
The liposomal nanoparticles (L3 & S1) were used to inhibit the growth of T. vaginalis. As seen in Figure 3, increasing the inhibitory effect of thymol as the concentration increased, the differences in the inhibitory effect of thymol against T. vaginalis were significant in most of the concentrations (P <0.01). This indicated the effect of raising the thymol concentration on the inhibition of the parasite growth. The IC50 for thymol was obtained at different times using the calculation formula, 10.022, 6.728, and 6.607 μg/ml at 24, 48, and 72 hours, respectively. See Figure 6.
Figure 3 compares the average growth inhibition percentage of T. vaginalis at different thymol concentrations for 24, 48, and 72 hours. Figure 4 demonstrates the rise in the inhibitory effect of thymol nanoparticles by increasing its concentration. The difference in the inhibitory effect of thymol nanoparticles on T. vaginalis was statistically significant at the 3.59 µg/ml concentration and above within 24 hours, and the 3.29 concentration and above within 48 hours. The inhibition was evident at all concentrations within 72 hours (P <0.01). The IC50 for thymol nanoparticles within 24, 48, and 72 hours was 64.202, 16.471, and 9.55 μg/ml, respectively. See Figure 6.
Figure 4 compares the mean growth inhibition of T. vaginalis at different concentrations of thymol nanoparticles during three time points of 24, 48, and 72 hours. Similar to other preparations, the effect of thymol liposomal form increased with raising the concentration. The difference in the inhibitory effect of the liposomal form of thymol on T. vaginalis was significant (P<0.01) at the thymol concentration of 2.99 μg/ml and above within 24 hours, and at all concentrations within 48 and 72 hours. See Figure 5. The IC50 concentrations for the liposomal thymol at 24, 48, and 72 hours were 11.86, 7.41, and 4.71µg/ml, respectively. See Figure 6.
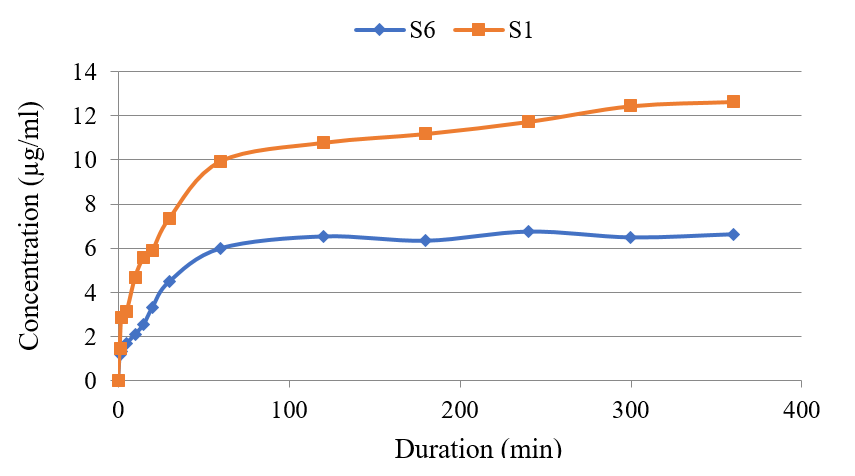
Figure 1. Comparison of thymol release from its nanoparticle form S1 and S6 formulations.
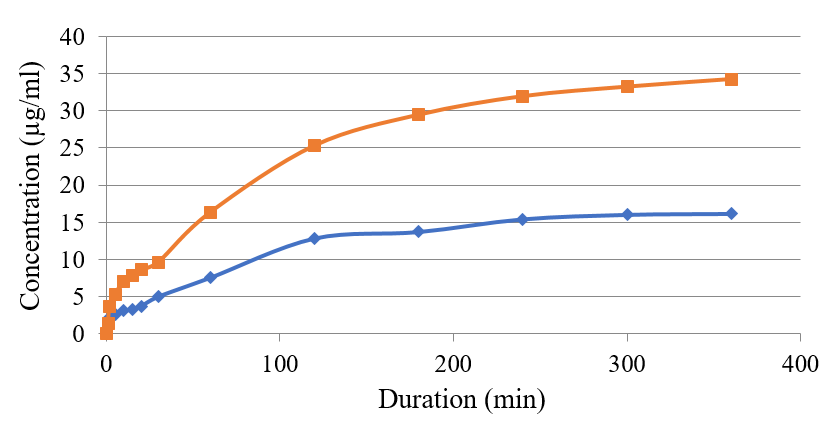
Figure 2. Comparing thymol release concentration from its liposomal form (L3 & L6). The red line is phosphatidylcholine; the blue line is Tween-20.

Figure 3. Comparison of the average growth inhibition percentage of T. vaginalis at varying concentrations of thymol over three durations, 24, 48, and 72 hours.
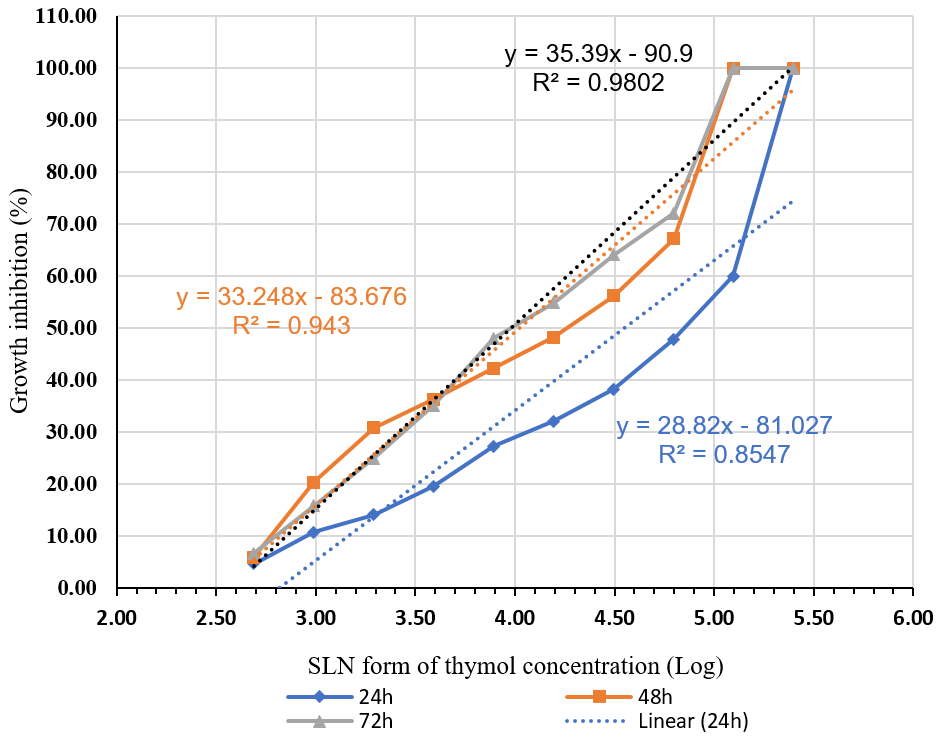
Figure 4. Comparison of the mean growth inhibition of T. vaginalis at varying concentrations of thymol nanoparticles over three durations: 24, 48, and 72 hours.
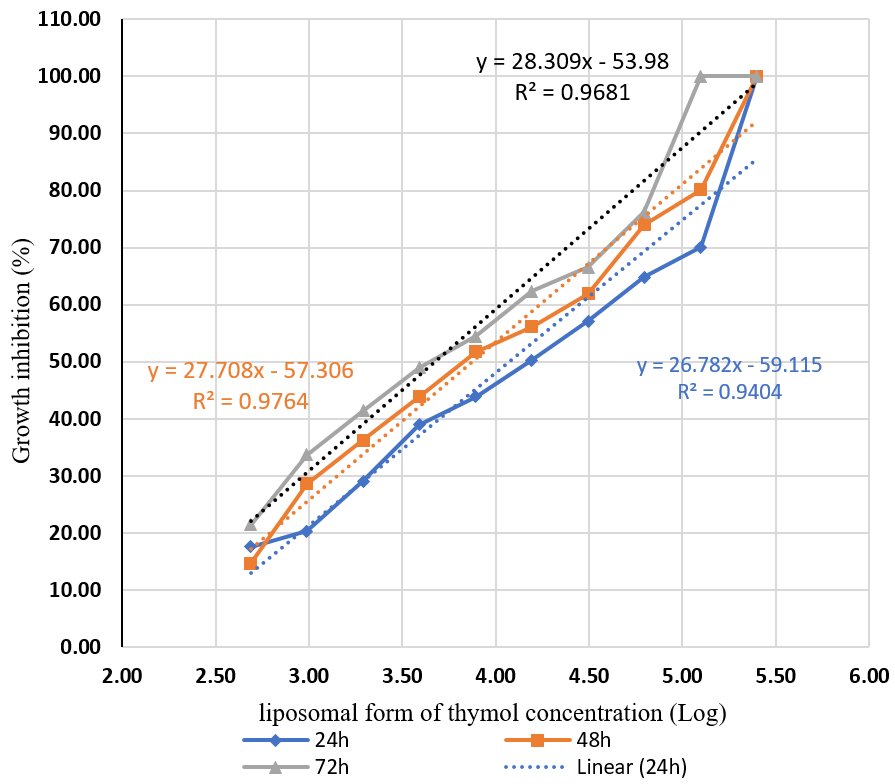
Figure 5. Comparison of the mean percentage of growth inhibition of T. vaginalis at varying concentrations of the liposomal thymol over three durations: 24, 48, and 72 hours.
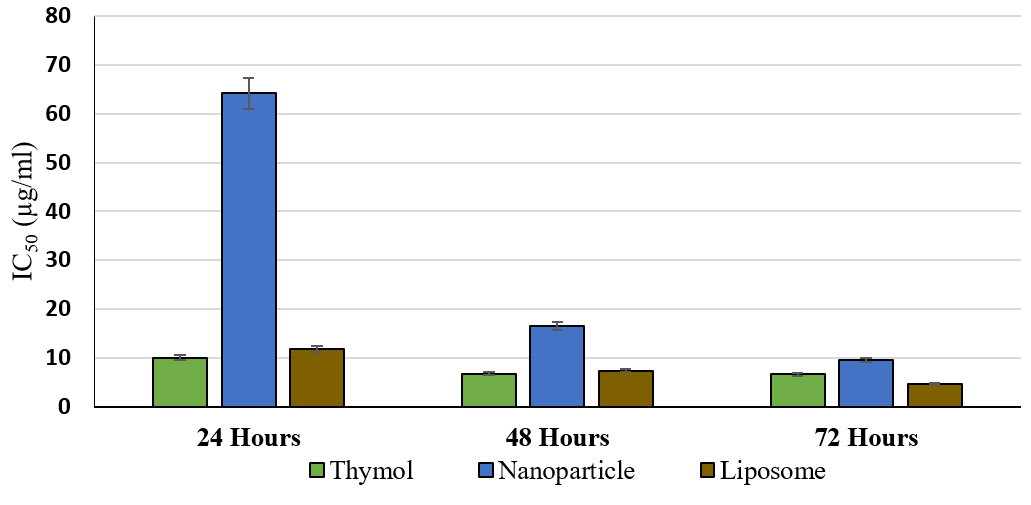
Figure 6. Comparison of the IC50 efficacy of thymol, and its nanoparticle and liposomal forms against T. vaginalis at varying treatment durations.
Figure 5 compares the mean percentage of growth inhibition of T. vaginalis at different concentrations of the thymol liposomal form during three time points of 24, 48, and 72 hours. The results demonstrated that the average percentage of growth inhibition did not differ significantly by increasing the exposure time from 24 to 48 and 48 to 72 hours. In addition, the average rate of growth inhibition of the pure thymol at different time points was almost similar compared to those of the nanoparticle and liposomal forms. See Figure 6. As reflected in Figure 6, the efficacy (IC50) of pure thymol is compared to those for the nanoparticles, and liposomal forms against T. vaginalis at different exposure times.
Discussion
Herbal medicines are inexpensive due to ample availability of raw materials and greater patient acceptance (20), hence, the popularity of plants products as alternative therapies in today’s healthcare (21). Although some herbal products can inhibit the growth of one or more protozoan species, very few of them kill the organisms (22). Metronidazole is an anti-protozoan drug by breaking the DNA strands; while the mechanism of thymol action against parasites is known to permeate and disrupt cell membranes and the surface cations, thus inhibiting the vital parasite activities (23).
Metronidazole is the suggested drug of choice for trichomoniasis, although it has its side effect and lead to microbial resistance (24). Patients with trichomoniasis sometimes have to undergo a multi-stage, long-term treatment due to recurrence of the infection. In such cases, the application of an alternative agent, such as thymol are known to be beneficial to patients (25, 26) This justifies the increased popularity and search for effective natural alternative medicines (26). Therefore, it seems that products from thymol-containing plants are likely to be appropriate treatment choices in these cases. However, further clinical studies are warranted to establish the efficacy of the plants products. In this context, studies have already shown the anti-trichomoniasis effect of thymol-containing compounds. Thymol is also effective in relieving itching in the genital area, which increases the importance of its use in such clinical cases (27, 28).
In a study by Golmakani, et al., the effect of a vaginal cream containing thymol was evaluated versus that of Metronidazole (29). The results demonstrated that the success rate of a wet smear-based treatment was 51.9% in the thyme group versus 88.9% in the Metronidazole counterpart (30). The results of the present study demonstrate that the three plant products; i.e., pure thymol, the nanoparticle and liposomal forms effectively inhibit the growth of Trichomonas, the inhibitory effect of which increases by raising its concentration. Although the inhibitory effect increased slightly over longer treatment periods, this effect was not significant on the parasite inhibition within the duration used in the current study. It was also shown that the average percentage of growth inhibition did not differ significantly by increasing the treatment duration from 24 to 48 and to 72 hours. In addition, the average inhibition rate of the parasite’s growth by thymol alone over different treatment periods with the nanoparticle and liposomal forms was almost the same.
It was also revealed that the three forms of thymol used at higher concentrations produced similar inhibition in the parasites compared to that of Metronidazole at 65 μg/ml. The liposomal formulation caused 100% growth inhibition of the parasites when the treatment time increased to 72 hours, which was significantly better than that of thymol alone. Based on the findings of one study by Wattanasatcha (31), nanoparticles and nanoliposomes did not seem to significantly affect the growth inhibition of the parasite relative to thymol alone despite the additional cost and difficulty for their preparation (31). This study evaluated the anti-bacterial effect of thymol nanoparticles, and showed that they had lower MIC and MBC than pure thymol extract against Staphylococcus aureus, E-Coli, and Pseudomonas aeruginosa. This study also showed that the use of nanocapsule form improved the duration of drug effect, i.e., the nanoparticle form suppressed bacterial life up to 3 months while the extract only suppressed it for 2 to 4 weeks (31).
In another study (32), herbs containing thymol and carvacrol have been used, and demonstrated that thymol effectively inhibited Trichomonas parasites experimentally at two concentrations of 0.1 and 0.01% as did Metronidazole. Furthermore, similar to the present study findings, increasing the exposure period from 24 to 48 and 72 hours did not significantly affect the antiparasitic power of the thyme extract. It seems that the liposomal and nanoparticle forms of thymol can stabilize and increase the duration of drug effect. The efficacy of the liposomal was more than the thymol alone within72 hours, as evident by the current study's findings. However, we found no significant differences among the three forms, i.e., thymol alone, nanoparticle form, and liposomal thymol at different concentrations. Pivetta, et al. also showed that thymol nanoparticles and encapsulation effectively released the active ingredient, which increased the stability of the drug and reduced its toxicity (33, 34). Therefore, it appears that the nanoparticle and liposomal and encapsulation forms of thymol, can improve the drug stability.
In line with the present study, Ardestani, et al. showed that thymol at 4000 μg/ml within 24 hours inhibited the growth of T. vaginalis by 91%. In that study, the IC50 values for thymol over 24 and 48 hours were 195.9 and 91.85 µg/ml, respectively. Similar to our findings, Ardestani, et al.’s study showed that the effect of thymol was dependent on its concentration and treatment duration. Although the impact of increasing the treatment duration from 24 to 48 hours was small, the average percentage of growth inhibition by increasing the thymol concentration from 125 to 4000 µg/ml had a significant impact (35).
Conclusions
Based on our findings, the percent inhibition of T. vaginalis by pure thymol versus its nanoliposome and nanoparticle forms depended on the duration and the concentration. Thymol and its nanoliposome form showed a lower inhibitory effect (IC50) on T. vaginalis than that of the thymol nanoparticles over 24 or 48 hours of treatment. However, the efficacy and the IC50 values of the three thymol formulations did not significantly differ after 72 hours of treatment.
Ethical Considerations
This study was approved by the Ethics Committee of Shahid Sadoughi University of Medical Sciences, Yazd, Iran. (Code: IR.SSU.MEDICINE.REC.1398.180).
Compliance with Ethical Guidelines
No animals were used in this study. The T. vaginalis strain was used in the culture medium. During the study, ample hygienic guidelines were used to prevent the spread of this protozoan to humans and the laboratory environment. At the completion of the study, all of the remaining protozoan samples were discarded safely with other infectious wastes at the isolated disposal area of the School of Pharmacy for regular disposal.
Funding
This study was funded solely by the authors.
Authors’ Contributions
Conceptualization, supervision, and methodology: MZ and FM; Investigation: SJ, MZ, FM & VR; Writing the original draft of the manuscript: SJ, HG; Review & editing: HG, MZ; Data collection: SJ, FM and VR; Data analysis: SJ and MZ; Funding Administration: MZ and FM.
Conflict of Interest
The authors declare no known competing financial interests or personal relationships that could have influenced the work reported in this article.
Acknowledgment
This work was supported for the laboratory space and equipment by the School of Pharmacy, Shahid Sadoughi University of Medical Sciences, Yazd, Iran.
References
1. Newman L, Rowley J, Vander Hoorn S, Wijesooriya NS, Unemo M, Low N, et al. Global Estimates of the Prevalence and Incidence of Four Curable Sexually Transmitted Infections in 2012 Based on Systematic Review and Global Reporting. PloS one. 2015; 10(12):e0143304. [doi: 10.1371/journal.pone.0143304] [pmid: 26646541]
2. Geelen TH, Hoebe CJ, Dirks A, Dukers-Muijrers NH, van Bergen JE, Wolffs PF. Low positivity rate after systematic screening for Trichomonas vaginalis in three patient cohorts from general practitioners, STI clinic and a national population-based chlamydia screening study. Sexually transmitted infections. 2013; 89(6):532-4. [doi: 10.1136/sextrans-2012-051010] [pmid: 23821769]
3. McClelland RS, Sangare L, Hassan WM, Lavreys L, Mandaliya K, Kiarie J, et al. Infection with Trichomonas vaginalis increases the risk of HIV-1 acquisition. The Journal of infectious diseases. 2007; 195(5):698-702. [doi: 10.1086/511278] [pmid: 17262712]
4. Van Der Pol B, Kwok C, Pierre-Louis B, Rinaldi A, Salata RA, Chen PL, et al. Trichomonas vaginalis infection and human immunodeficiency virus acquisition in African women. The Journal of infectious diseases. 2008; 197(4):548-54. [doi: 10.1086/526496] [pmid: 18275275]
5. Shew ML. Association of condom use, sexual behaviors, and sexually transmitted infections with the duration of genital human papillomavirus infection among adolescent women. Arch Pediatr Adol Med. 2006; 160(2):151-6. [doi: 10.1001/archpedi.160.2.156]
6. van Schalkwyk J, Yudin MH, Infectious Disease C. Vulvovaginitis: screening for and management of trichomoniasis, vulvovaginal candidiasis, and bacterial vaginosis. Journal of obstetrics and gynaecology Canada : JOGC = Journal d'obstetrique et gynecologie du Canada : JOGC. 2015; 37(3):266-74. [doi: 10.1016/S1701-2163(15)30316-9] [pmid: 26001874]
7. Hezarjaribi HZ, Fakhar M, Shokri A, Teshnizi SH, Sadough A, Taghavi M. Trichomonas vaginalis infection among Iranian general population of women: a systematic review and meta-analysis. Parasitology research. 2015; 114(4):1291-300. [doi: 10.1007/s00436-015-4393-3] [pmid: 25732256]
8. Kissinger P, Amedee A, Clark RA, Dumestre J, Theall KP, Myers L, et al. Trichomonas vaginalis treatment reduces vaginal HIV-1 shedding. Sexually transmitted diseases. 2009; 36(1):11-6. [doi: 10.1097/OLQ.0b013e318186decf] [pmid: 19008776]
9. Petrin D, Delgaty K, Bhatt R, Garber G. Clinical and microbiological aspects of Trichomonas vaginalis. Clinical microbiology reviews. 1998; 11(2):300-17. [doi: 10.1128/CMR.11.2.300] [pmid: 9564565]
10. Schmid G. Prevalence of metronidazole-resistant Trichomonas vaginalis in a gynecology clinic. J Reproduct Med. 2001; 46(6):545-9. [doi: 10.1097/00006254-200111000-00015]
11. Hager WD. Treatment of metronidazole-resistant Trichomonas vaginalis with tinidazole: case reports of three patients. Sexually transmitted diseases. 2004; 31(6):343-5. [doi: 10.1097/00007435-200406000-00004] [pmid: 15167642]
12. Connor TH. The contribution of metronidazole and two metabolites to the mutagenic activity detected in urine of treated humans and mice. Cancer Res. 1977; 37(2):629-33.
13. Ghafari S. Plants used to treat "tabe rebá"(malaria like fever) in Iranian traditional medicine. Intl J Trad Herbal Med. 2013; 1(6):168-76.
14. Ultee A, Kets EP, Smid EJ. Mechanisms of action of carvacrol on the food-borne pathogen Bacillus cereus. Applied and environmental microbiology. 1999; 65(10):4606-10. [doi: 10.1128/AEM.65.10.4606-4610.1999] [pmid: 10508096]
15. Donsì F. Nanoencapsulation of essential oils to enhance their antimicrobial activity in foods. LWT Food Sci Technol. 2011; 44(9):1908-14. [doi: 10.1016/j.lwt.2011.03.003]
16. Wu Y, Luo Y, Wang Q. Antioxidant and antimicrobial properties of essential oils encapsulated in zein nanoparticles prepared by liquid-liquid dispersion method. LWT Food Sci Technol. 2012; 48(2):283-90. [doi: 10.1016/j.lwt.2012.03.027]
17. Cowan MM. Plant products as antimicrobial agents. Clinical microbiology reviews. 1999; 12(4):564-82. [doi: 10.1128/CMR.12.4.564] [pmid: 10515903]
18. Groll AH, Rijnders BJA, Walsh TJ, Adler-Moore J, Lewis RE, Bruggemann RJM. Clinical Pharmacokinetics, Pharmacodynamics, Safety and Efficacy of Liposomal Amphotericin B. Clinical infectious diseases : an official publication of the Infectious Diseases Society of America. 2019; 68(Suppl 4):S260-S74. [doi: 10.1093/cid/ciz076] [pmid: 31222253]
19. Ramezani V. Formulation and optimization of transfersome containing minoxidil and caffeine. J Drug Deliver Sci Tech. 2018; 44:129-35. [doi: 10.1016/j.jddst.2017.12.003]
20. Hashemi N, Ommi D, Kheyri P, Khamesipour F, Setzer WN, Benchimol M. A review study on the anti-trichomonas activities of medicinal plants. International journal for parasitology Drugs and drug resistance. 2021; 15:92-104. [doi: 10.1016/j.ijpddr.2021.01.002] [pmid: 33610966]
21. Mehriardestani M, Aliahmadi A, Toliat T, Rahimi R. Medicinal plants and their isolated compounds showing anti-Trichomonas vaginalis- activity. Biomedicine & pharmacotherapy = Biomedecine & pharmacotherapie. 2017; 88:885-93. [doi: 10.1016/j.biopha.2017.01.149] [pmid: 28178618]
22. Morteza-Semnani K, Azadbakht M, Hooshmand A. Chemical composition of the essential oils from the flowers and leaves of Ajuga reptans. Chem Natur Compound. 2018; 54(2):375-6. [doi: 10.1007/s10600-018-2352-9]
23. Howe K, Kissinger PJ. Single-Dose Compared With Multidose Metronidazole for the Treatment of Trichomoniasis in Women: A Meta-Analysis. Sexually transmitted diseases. 2017; 44(1):29-34. [doi: 10.1097/OLQ.0000000000000537] [pmid: 27898571]
24. Afarnezhad F. Comparison of the percentage of medical success for Phytovagex vaginal suppository and Metronidazole oral tablet in women with bacterial vaginosis. Iran J Obstet Gynecol Infertil. 2017; 20(3):29-39.
25. Seña AC, Bachmann LH, Hobbs MM. Persistent and recurrent Trichomonas vaginalis infections: epidemiology, treatment and management considerations. Expert Rev Anti Infect Ther. 2014; 12(6):673-85.
26. Corns CM. Herbal remedies and clinical biochemistry. Annals of clinical biochemistry. 2003; 40(Pt 5):489-507. [doi: 10.1258/000456303322326407] [pmid: 14503986]
27. Goudarzi M. Antibacterial effects of aqueous and alcoholic extracts of thyme on entrohemorrhagic Escherichia coli. Yafteh. 2006; 8:63-9.
28. Karimian Z, Keramat A. Hot flashes of menopause and herbal medicine in Iran: a systematic review. Iran J Obstet Gynec Infertil. 2014; 17(111):1-11.
29. Golmakani N. Comparing the effect of Zataria multiflora vaginal cream with metronidazole oral pills in the treatment of Trichomonas Vaginitis. J Nurs Midwife Mashhad. 2009; 9:81-8.
30. Ziaiye H. Effect of methanolic extracts of Artemisia aucheri Boiss, Zataria multiflora Boiss and Myrtus communis L. on Trichomonas vaginalis (In Vitro). J Gorgan Univ Med Sci. 2006; 8(1):34-8.
31. Wattanasatcha A, Rengpipat S, Wanichwecharungruang S. Thymol nanospheres as an effective anti-bacterial agent. International journal of pharmaceutics. 2012; 434(1-2):360-5. [doi: 10.1016/j.ijpharm.2012.06.017] [pmid: 22698863]
32. Azadbakht M. Effect of essential oils of Artemisia. Zataria and Myrtus on Trichomonas vaginalis. J Med Plant. 2003; 2(8):35-40.
33. Wingard JR, White MH, Anaissie E, Raffalli J, Goodman J, Arrieta A, et al. A randomized, double-blind comparative trial evaluating the safety of liposomal amphotericin B versus amphotericin B lipid complex in the empirical treatment of febrile neutropenia. L Amph/ABLC Collaborative Study Group. Clinical infectious diseases : an official publication of the Infectious Diseases Society of America. 2000; 31(5):1155-63. [doi: 10.1086/317451] [pmid: 11073745]
34. Pivetta TP. Development of nanoparticles from natural lipids for topical delivery of thymol: Investigation of its anti-inflammatory properties. Colloid Surfaces B: Biointerfaces. 2018; 164:281-90.
35. Ardestani MM, Aliahmadi A, Toliat T, Dalimi A, Momeni Z, Rahimi R. Evaluation of Antimicrobial Activity of Trachyspermum ammi (L.) Sprague essential oil and its active constituent, thymol, against vaginal pathogens. Trad Integr Med. 2020; 5(2):49-58.
Type of Study:
Research |
Subject:
General