Introduction
The application of natural products from plant sources in the management of several ailments is not a contemporary practice in modern healthcare. However, such products have been used for many years as tranquilizers (reserpine), antimalarials (quinine, artemisinin) cardiac stimulants (digitoxin), antiretrovirals, and calanolides A and B [1].
Malaria is one of the major threats to global healthcare. Every year, approximately 300-500 million acute infections and three million deaths arise from this disease in over 90 countries globally where malaria is prevalent [2]. It’s a recurring disease, with pregnant women and children below the age of 5 being the most vulnerable people [3]. In certain epidemiological occasions, malaria has high morbidity and mortality potentials, and it can be devastating with an urgent need to combat it.
One of the issues in combating malaria is the rapidly evolving and proliferation of the parasite resistance to otherwise effective drugs that are currently used [4]. Plasmodium species have greatly relegated a number of well-known anti-malarial agents today because of the ability to offer resistance to these drugs. Considering that there is no prospect for a potent vaccine, it is important to explore potential compounds, mostly of plant origins, to combat malarial infection [5]. This explains the wide-spread and increasing worldwide use of natural plant products, especially in the third world and developing nations.
A variety of compounds from plant origins has made them major candidates for developing bioactive therapeutic agents [6]. For example, quinine, extracted from Cinchona ledgeriana (Rubiaceae) has become a malaria treatment agent for more than a century. In the same way, sesquiterpene lactone (artemisinin) has been isolated from Artemisia annua (an antipyretic herb in China) and is recently gaining popularity for its anti-malarial property [7].
The hope for new and effective generation of anti-malarial drugs is hinged upon the screening of herbal drugs known for treating this disease traditionally. Usman et al. [8] states that more than 80% of human population use plants as basic treatments at some point in their lives [8, 9].
The most common drugs for treating pain and inflammatory conditions are opioids and non-steroidal anti-inflammatory drugs (NSAIDs) [10]. However, these drugs have adverse effects [11]. Therefore, the investigation for safer analgesics as new therapies is imperative. In traditional medicine, one of the common problems for using crude plant extracts is lack of proper evaluation of their toxicological profiles. In this context, the potential side effects of using crude extracts can lead to organ and tissue damages [12].
Therefore, the screening of medicinal plants known for their anti-malarial properties is gaining popularity. Newbouldia laevis (N. laevis) and Crateva adansonii (C. adansonii) are few of such plants that are suspected in Nigeria for their effects against malaria related fever [13, 14]. This study was planned to investigate the N. laevis and C. adansonii leaf extracts for their anti-plasmodial activities. This is an important step to uncover their potentials to become natural resources toward new anti-malarial drug development.
Materials and Methods
Reagents and chemicals: All of the reagents and chemicals used in this study were of analytical grades, and obtained from Sigma Aldrich Chemicals (St. Louise, MO, USA).
Plant Samples: Fresh green N. laevis and C. adansonii leaves were collected from Baddegi, Niger State, Nigeria. They were identified and authenticated by the Biological Science Department of the Federal University of Technology, Minna, Niger State, Nigeria.
Experimental Animals: Healthy 6-week old Swiss albino mice (male and female) weighing 20-30 g, and Wistar rats weighing 180-200 g were used in this study. The 20 mice that were used in this study were divided into five groups of four mice each. One group was assigned as the control (with 20 ml/kg normal saline). They were acquired from National Institute of Pharmaceutical Research and Development in Abuja, Nigeria.
Malaria parasites: Chloroquine-sensitive Plasmodium berghei (NK-65 strain) was acquired from the National Institute of Pharmaceutical Research and Development in Abuja, Nigeria. It was maintained in healthy laboratory mice by serial-passage.
Inoculation of animals: EDTA-treated bottles were used to collect parasitized erythrocytes from a donor-mouse, which had been infected with the parasite earlier. Blood samples were collected and subsequently diluted with normal saline from the jugular vein of the infected mouse, into an EDTA-treated bottle. A 0.2 ml of the infected blood suspension was used to inoculate each of the experimental mice intraperitoneally [15].
Preparation of crude extracts: The extraction methods selected in this study was on the basis of the yield obtained. Leaf samples (40 g, total) were pre-dried with air, ground and extracted sequentially in an exhaustive manner for 48 hr with 400 ml of hexane (Sigma-Aldrich, UK) followed by ethylacetate and methanol. The material obtained subsequently was filtered with muslin cloth. After filtration, the recovered solvents were lyophilized under vacuum in a rotary evaporator. The green colored pastes were subsequently collected and weighed before further analysis.
Animal management: A total of 20 mice divided into five groups of four each were used in this study. They were placed in a comfortable space furnished with standard environmental conditions and had free access to commercially available pellet food and drinking water. The experiments followed the universally recognized standards for laboratory animal use and care by the Canadian Council on Animal Care guidelines and review protocol [16].
Safe dose and acute toxicity of the extracts: We used Lorke’s methodology to determine the safe dose and acute toxicity (LD50) levels. The rodents were administered per group with the extracts of N. laevis at 100, 200, 300, 400 or 500 mg/kg body weight doses intraperitoneally. The leaf extract of C. adansonii was also administered to five groups of four mice intraperitoneally at doses of 600, 800, 1600, 3000 or 3200 mg/kg. The medium for dissolving the extracts was DMSO. The control group was separately administered with 20 ml/kg of normal saline. The mice were then observed for over 72 h, and the clinical signs and mortality were documented. The LD50 of the extracts were determined from the plot of the intercept of percent mortality (y-axis) and dosages (x-axis) [17].
Anti-plasmodial screening: Before starting the study, the mice were screened microscopically, using blood smears from the tails. This step was done to exclude the likelihood of animals concealing rodent Plasmodium species. The preliminary anti-plasmodial effects of different fractions of the extracts of N. laevis and C. adansonii in P. berghei infected mice were also carried out. This was an essential step to obtain the fraction with the highest anti-parasite activity.
The experiment: Fidock et al.’s method for probing the anti-plasmodial activity of plant extracts was used in mice infected with P. berghei [15]. DMSO was the medium for dissolving the plant extracts. It served as a transport medium for the extract delivery to the animals. This method involved treating the animals with the plant extracts three days after inoculating the mice with the parasite. One of the infected mice that served as the donor was then anaesthetized with chloroform. Blood samples were taken from the donor through cardiac puncture, using sterile and heparinized syringes and needles.
The blood samples were then diluted using normal saline to the point that 0.2 ml had about 10 million infected RBCs. The twenty uninfected mice were subsequently inoculated each with 0.2 ml of the diluted blood sample intraperitoneally. The mice were administered with the extracts intraperitoneally once daily for five consecutive days (day 3 to day 7).
Group 1 was treated with 200 mg/kg of the methanol extract of N. laevis. Group 2 was administered 600 mg/kg of the ethylacetate extract of C. adansonii while Group 3 was administered 100 mg/kg (1:1w/w) of the combined extracts of N. laevis and C. adansonii. Group 4 received a similar trial where they received chloroquine (5 mg/kg) as a reference. Group 5 was treated with 20ml/kg of normal saline and was regarded as the negative control. Thin blood smears were prepared from the tail vein starting on day 3 and subsequently on alternate days. The blood smears were then stained, using 4% Giemsa (pH 7.2) for 45 minutes and examined by light microscopy. Five optic fields were observed on each slide and the numbers of infected RBCs were recorded. Subsequently, the mean and percent activity or parasitemia suppression were determined. The mean parasitemia per microscopic field (y-axis) and the number of days (x-axis) was plotted against each other.
Parasitemia inhibition: The percentage of parasites inhibited for every dose was calculated based on the following formula 1:
Mean Survival Period (MSP): The death rate among the animals was documented every day. We recorded the days from the onset of parasite inoculation to the time when the animals died in each group. The survival period in each group was calculated by dividing the mean survival time of the mice after infection by the time frame of 45 days (D0-D45) as follows (formula 2):
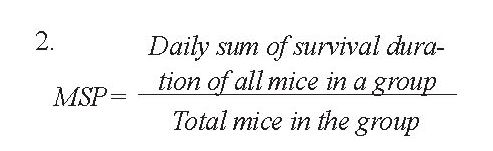
Changes in body weight: A sensitive digital balance (Scientech; Colorado, USA) was utilized to weigh each mice from each group before infection (day 0), during and after the treatments (15 and 30 days post treatment).
Packed Cell Volume: The Packed Cell Volume (PCV) was determined to monitor changes in the levels of the RBCs during the study according to an established method [18]. The tip of the mice tails was incised to collect the blood samples into heparinized capillary tubes and sealed with plasticine, a sealing agent. The capillary tubes were centrifuged at 11,000 rpm for 5 minutes. The packed cell volume was then determined using a micro hemotocrit reader.
Statistical Analyses: The statistical data were analyzed, using SPSS software v. 16. The outcomes were expressed in Means±SEM (Standard Errors of Means). Also, the Student’s t-test was utilized in the analysis of data among the groups. The results had at least a statistical significance level of P<0.05.
Results
Extract Yields: The extract yields from the two plant samples were not the same amount from the three different solvents used for extraction (n-hexane, ethylacetate and methanol). The ethylacetate extraction of C. adansonii gave the highest yield while the highest yield for N. laevis was obtained from methanol extraction. The activities of the two extracts were standardized on the basis of the preliminary results, and the most effective doses were then selected. From the methanol extraction, the highest yield for N. laevis was 1.021 g (2.55%) while the lowest yield for C. adansonii was 0.88 g (2.20%). The ethylacetate extraction of C. adansonii gave the highest yield of 1.53 g (3.83%) and lowest yield for N. laevis 0.688 g (1.72%). The n-hexane extraction of N. laevis gave 0.983 g (2.46%) and 1.04 g (2.60%) for C. adansonii.
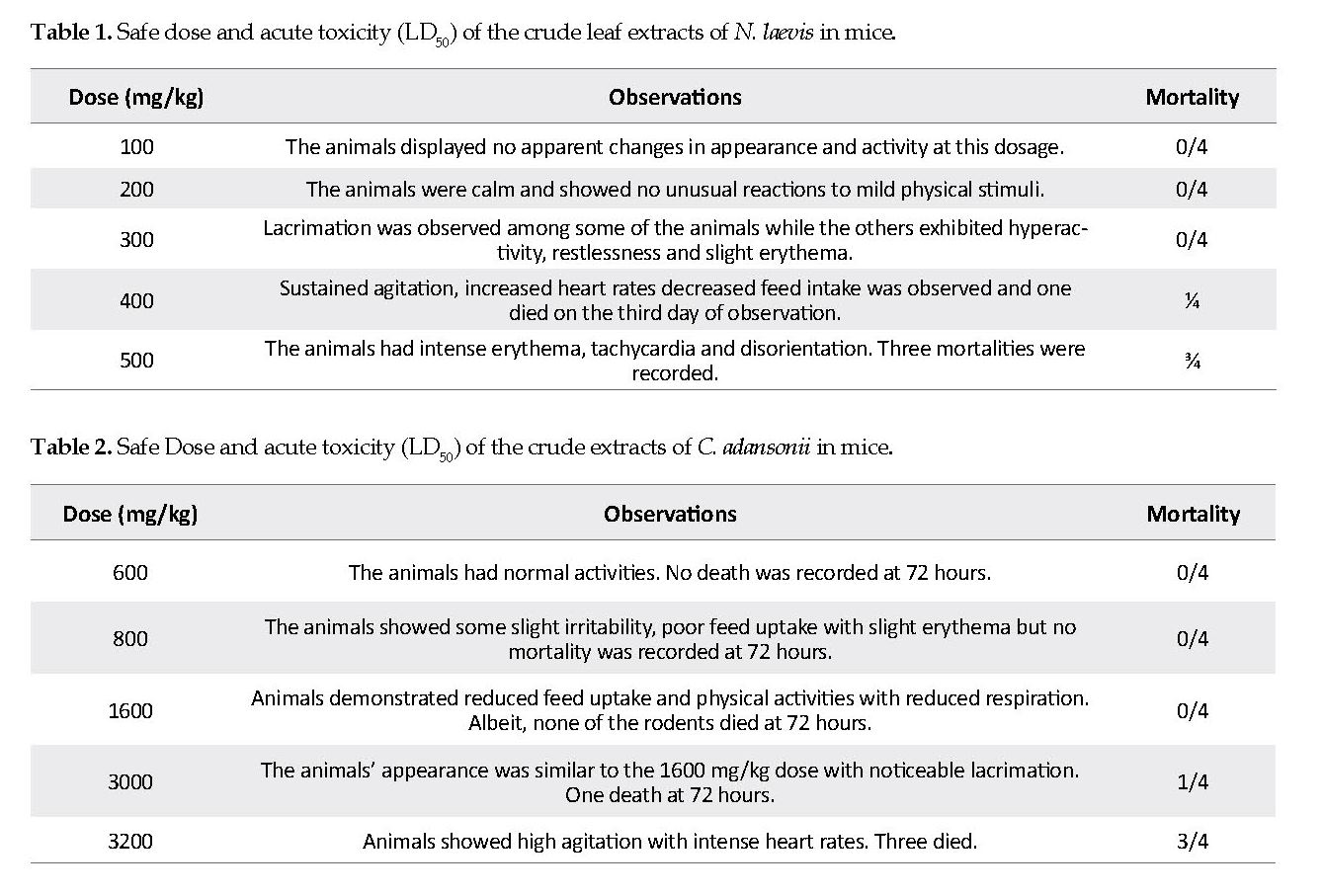
Safe dose and ld50 of the extracts: The safe intraperitoneal dose of the methanol extract of N. laevis in the mice was 200 mg/kg (Table 1). The doses between 100-200 mg/kg were safe and caused no adverse effects. The LD50 dose of the N. laevis extract was 471.43 mg/kg. The safe intraperitoneal dose of the extract of C. adansonii by ethylacetate method was 600 mg/kg (Table 2). The doses between 100-600 mg/kg were safe in the mice and caused no adverse effect, with the LD50 being 3,500 mg/kg.
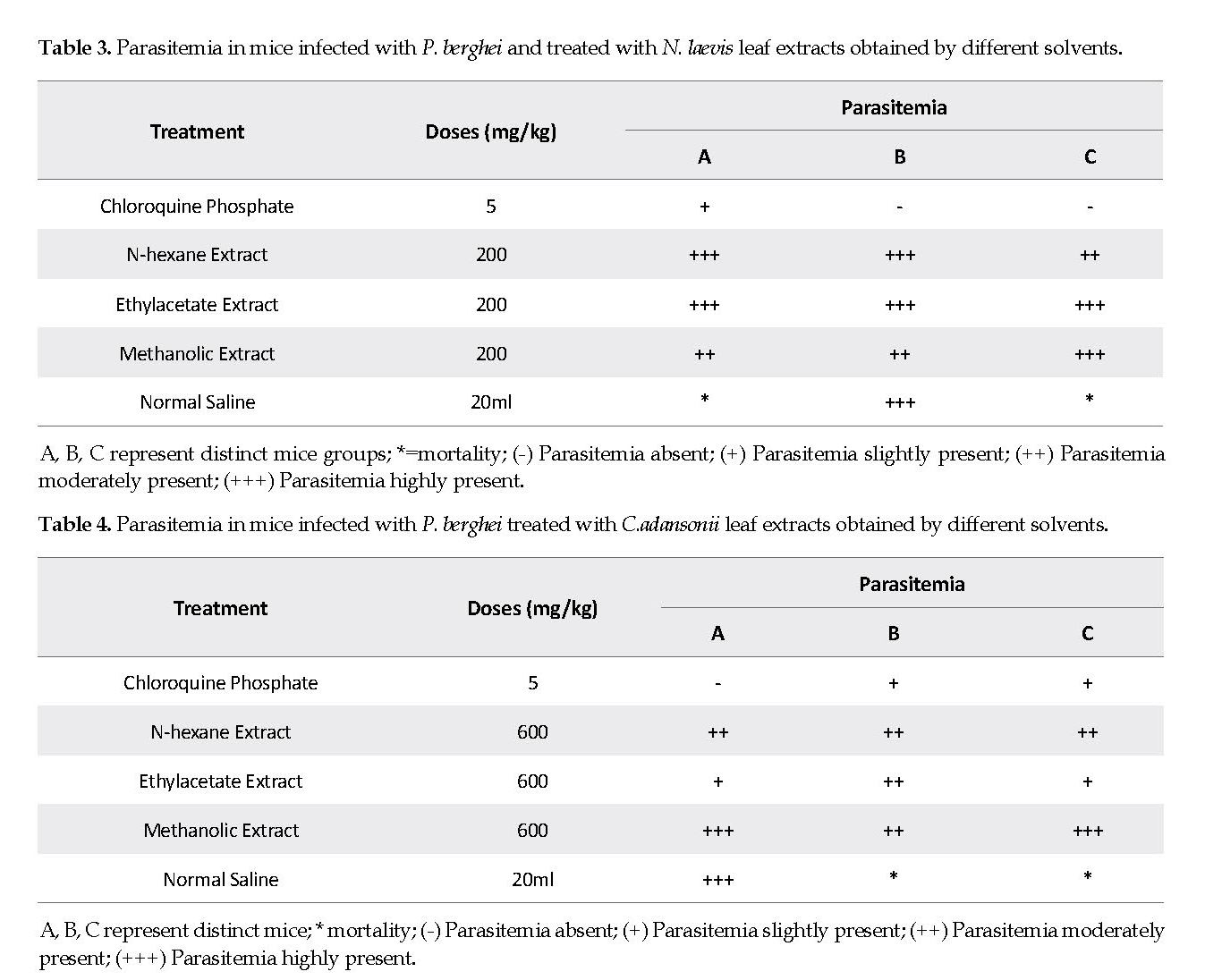
Effects of the extracts in infected mice: The preliminary anti-plasmodial screening results of the crude extracts of N. laevis and C. adansonii leaves are presented in Tables 3 and 4, respectively. The methanol extract of N. laevis showed a high suppression of the parasite, while the ethylacetate extract of C. adansonii had the best inhibitory effect on the parasite. However, the combination of two extracts was not as effective as the individual ones. The infected mice that were treated with normal saline did not survive the parasitemia.
Data obtained from the mice infected with P. berghei and treated with the extract of N. laevis is illustrated in Figure 1. The amount of parasite in the mice treated with standard chloroquine treatment decreased progressively from the 9th day (142.00±3.56 parasitemia per microscope field [ppmf]) to the 18th day (5.00±1.41 ppmf) and was totally cleared of it by the 21st day. The parasite count similarly decreased progressively in the mice treated with the methanol extract of N. laevis from the 9th day (138.40±4.83 ppmf) to the 18th day (85.20±5.99 ppmf).
However, the group treated with normal saline showed increased parasitemia level from 3rd to the 21st day after which all of the mice died. A significant change (P<0.05) in the parasitemia levels was observed by comparing the mice treated with the extract of N. laevis with those in the positive control groups treated with chloroquine only (Figure 1).
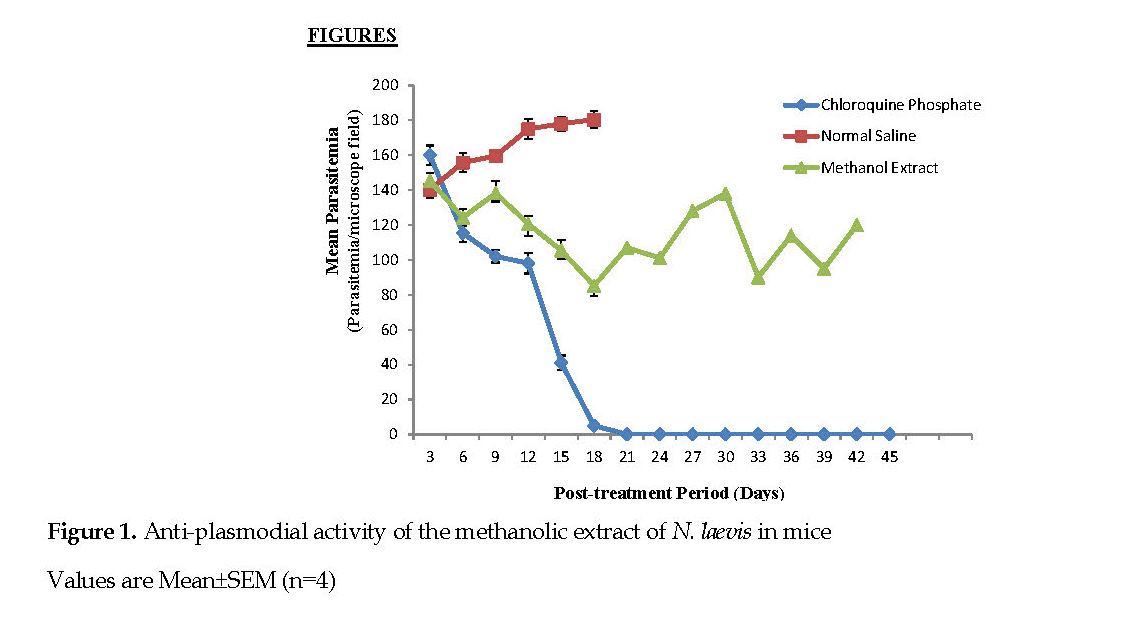
Figure 2 represents the significant reduction (P<0.05) in the parasite counts found in the group that received the ethylacetate extract of C. adansonii although, there was no total clearance of the parasites compared to that noted in the standard drug group. The highest and lowest parasite counts for this extract were 123.33±2.08 ppmf and 5.33±1.45 ppmf on the 9th and 45th days, respectively.
Figure 3 represents the anti-plasmodial activity of the mice infected with P. berghei in response to the combined extracts of N. laevis and C. adansonii. A progressive reduction in parasitemia was observed from the 6th day (140.88 ppmf) to the 21st day (92.33 ppmf) when all of the mice died. The normal saline group had the highest parasitemia value (160.88 ppmf) and all of them died on the on 21st day. The standard drug (chlo roquine) had the lowest parasite count (5.00±1.41 ppmf).
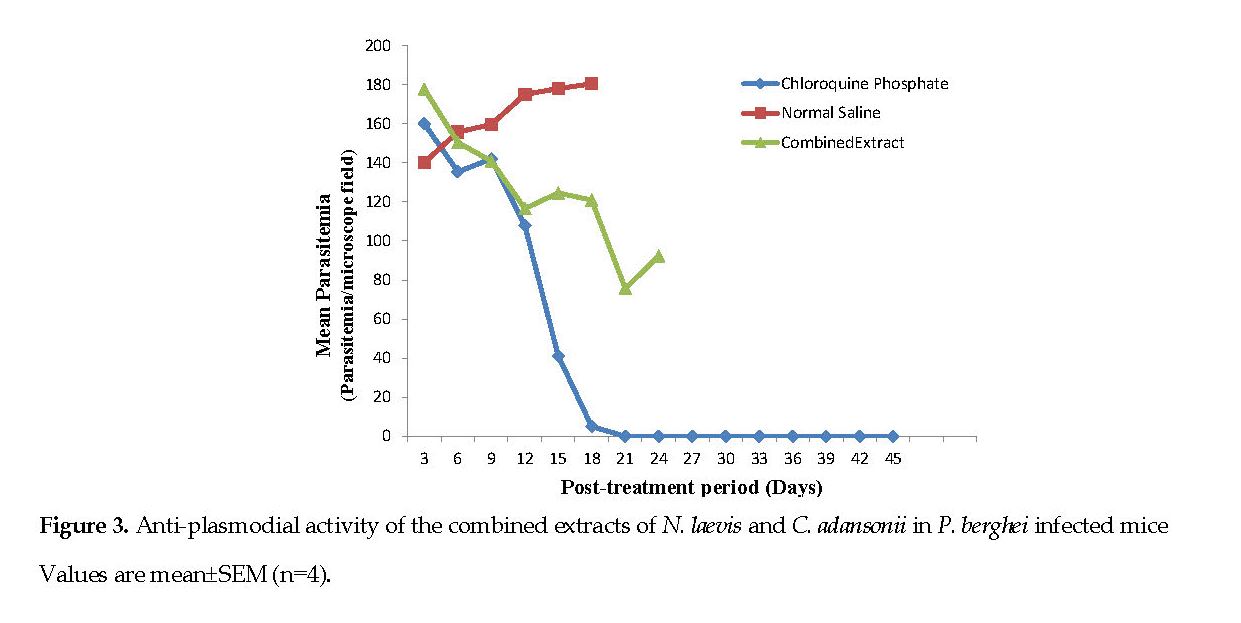
Table 5 presents the percentage of parasites inhibited by the treatments with the individual extract of N. laevis or C. adansonii or the combination of the two extracts (1:1w/w) in the infected mice. The mean parasites inhibition achieved by the standard drug (78.89±1.28%) was significantly different (P<0.05) from that of the extract of N. laevis (30.14±2.05%). The percent inhibition of extract of C. adansonii rendered a 61.35±1.41% parasites inhibition and was significantly different (P<0.05) from that induced by chloroquine. Treatment with the combined extracts caused a lower percentage of the parasite inhibition (24.23±0.86%) compared to that by either extract alone.
Mean Survival Period (MSP): The average endurance period of the infected mice that were treated with N. laevis or C. adansonii extract are shown in Table 6. The groups treated with the extracts of N. laevis or C. adansonii showed the highest MSP of 38.71days and 40.24 days, respectively. The MSP of the mice that received the equal combination of the extracts was 28.15 days. The mice in the positive control group had a MSP of 48.60 days, and those administered with normal saline showed the least MSP of 12.85 days.
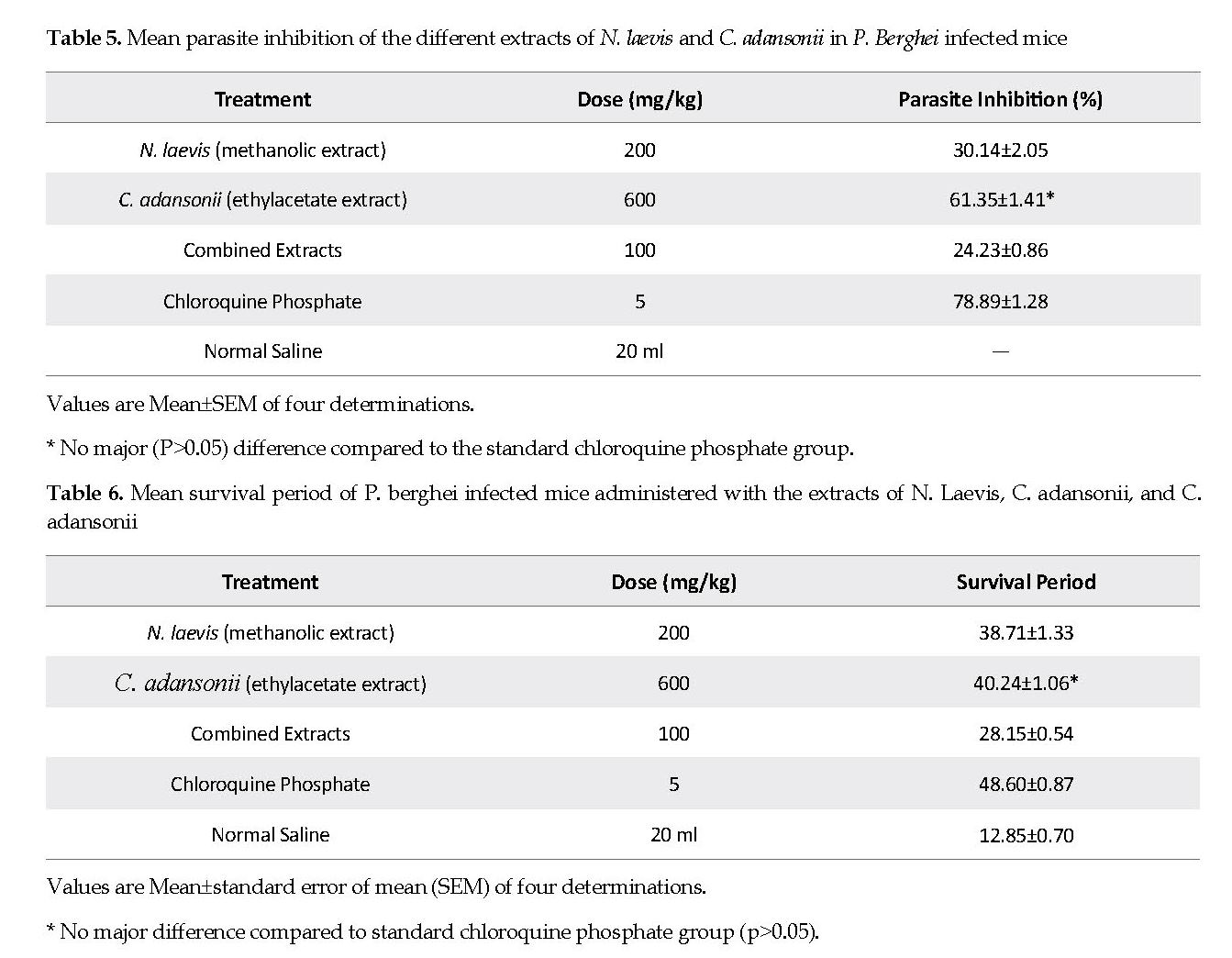
Changes in Body Weight: Variations in the average body weight of the infected mice, treated with one of the three different extracts are shown in Figures 4 and 5.
Weight reductions were observed in all groups after inoculation with the parasite. No major differences in the mean weight changes were observed in the mice treated with extract of either N. laevis or C. adansonii compared with that for the standard chloroquine group. The groups administered the combined extracts and the standard drug group progressively showed increases in weight beginning on the 3rd day to the 30th day after treatment.
The group treated with normal saline showed a weight loss beginning on the inoculation day and progressed until the 15th day post treatment (Figure 4).
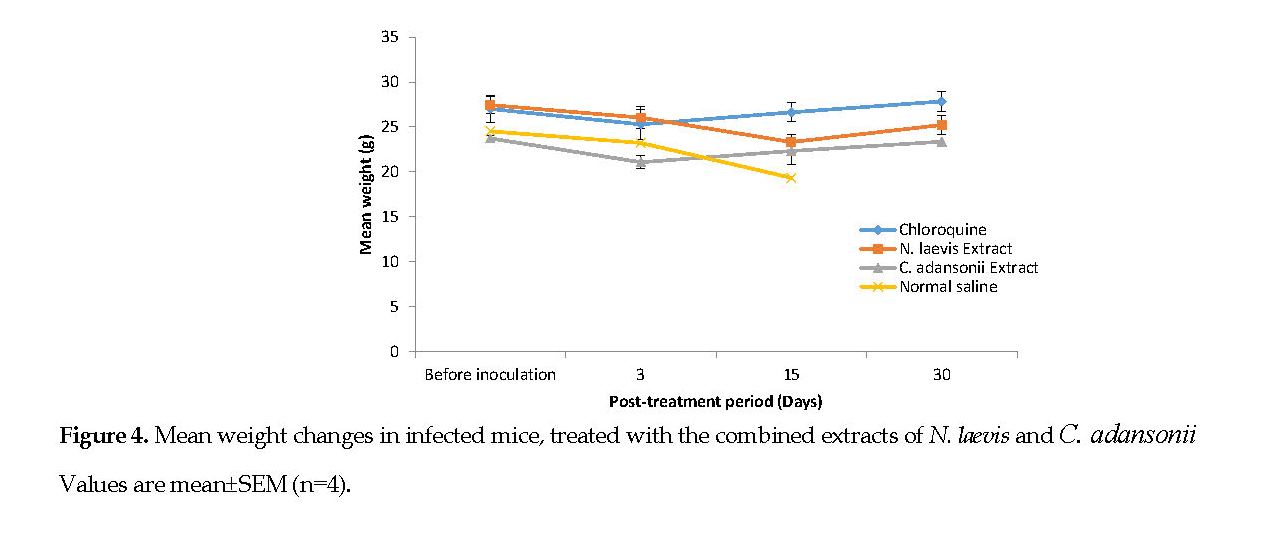
Changes in Packed Cell Volume (PCV): Figure 6 represents the mean PCV data documented for the infected mice, treated with the plant extracts. Figure 7 represents the mean PCV for the infected mice, treated with the combined extracts. After infecting the animals, a decline in the PCV was noted from the 3rd day of inoculation onward. Conversely, there was a progressive increase in the PCV in all treatment groups. On the 30th day post treatment, no major differences (P<0.05) in PCV of the mice treated with either extracts were noted although the two groups had clearly lower PCV’s than the standard drug group.
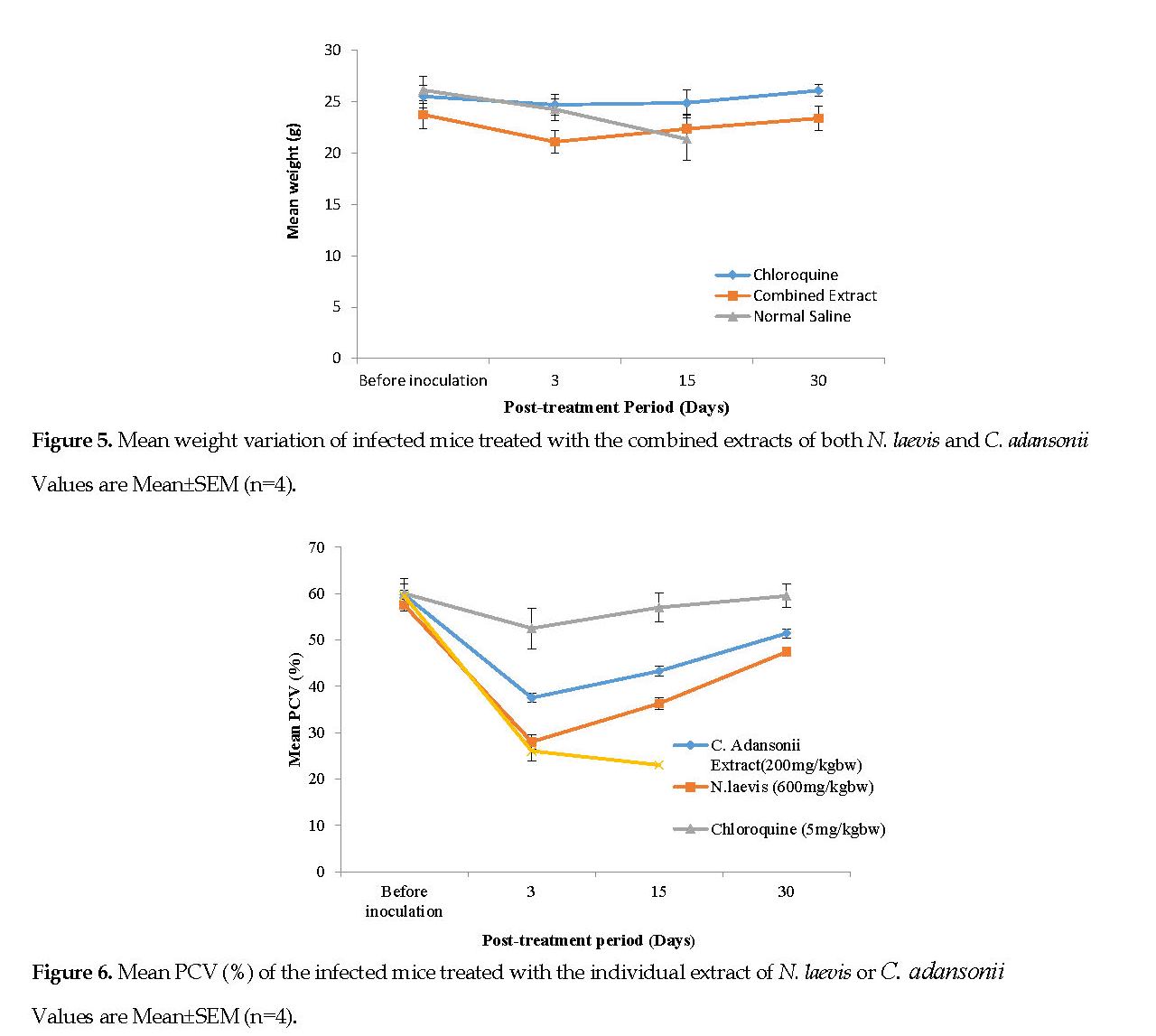
Discussion
Application of medicinal plants is popular in Africa and developing countries. In this context, researchers believe that medicinal plants are useful resources for the development of new therapeutic agents [19-21].
The safe dosage and LD50 results from the acute test of the N. laevis extract (Table 1) suggest the high tolerance of rats treated with it. The reduced toxicity of the N. laevis extract is likely due to the digestive processes occurring in the gastrointestinal tracts, where the extract is metabolized to less toxic compounds. However, the crude extract of N. laevis administered intraperitoneally at doses higher than 200 mg/kg tends to be unsafe. This concept also applies to other plant extracts with therapeutic potentials that are mostly safe, but some tend to be harmful especially at high doses [22].
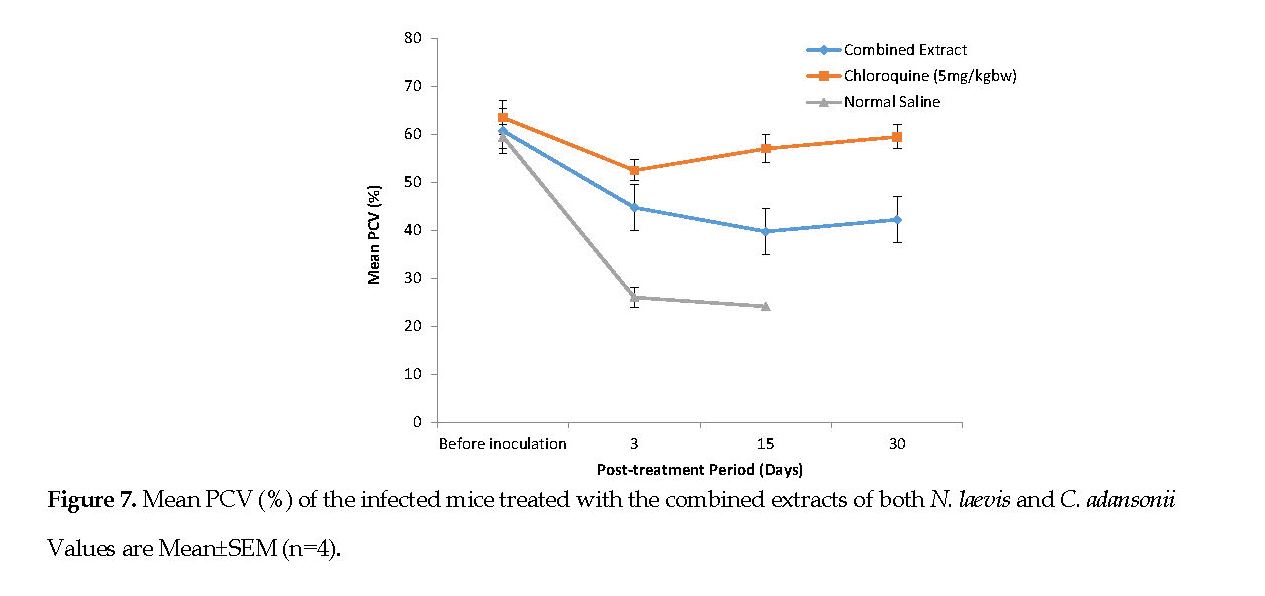
Table 2 presents the safe dose and LD50 of C. adansonii extract in the mice. It showed no acute toxicity and this justifies its wide application in traditional medicine. The high toxicity of certain medicinal plants limits their clinical use even though they are powerful pharmacologically. An example is simaroubaceae extract with an LD50 of 1.8 mg/kg [12]. Akanji et al., have shown that the aqueous extract of C. adansonii leaves at doses between 325 and 1300 mg/kg resulted in mild and reversible hematological and biochemical changes in the small intestine, liver and kidneys of experimental animals [23]. The high safety margin of this extract makes it a candidate for future drug development and research.
Our findings on the inhibitory activity of N. laevis extract on the parasite (Figure 1) are consistent with those reported by previous studies that used the extracts of Asparagus africanus, Withania somnifera [24], and Clerodendrum myricoides [25]. However, the parasitemia inhibition by the N. laevis extract was less than half of that induced by chloroquine, the standard drug (30.14% vs. 78.89%).
The marked suppression of P. berghei in the mice treated with the C. adansonii extract (Figure 2) implies its significant anti-plasmodial activity, which could be due to its bioactive components. Carvalho et al. have considered any compound that inhibits parasitemia greater than 30% as active [26]. The parasitemia inhibition achieved by the C. adansonii extract in our study was consistent with an earlier study on the extract of Azadirachta indica (52.32%) [27].
The high percentage of the parasite inhibition achieved by chloroquine may arise from the interruption of heme polymerization. It has been suggested that the crude plant extracts have better plasmodistatic than plasmodicidal properties since the crude bioactive components may need to be converted initially, and this time lag allows for continued parasitic proliferation [28]. The significant reduction (P<0.05) in the percentage of parasite inhibition (24.23%) observed for the group treated with the combined extracts compared to that for the standard drug group and the individual plant extracts (Table 5) may be explained by the interplay between the bioactive ingredients of the individual plant extract leading to the formation of an inactive complex. According to Fidock et al., the availability of the active components in sufficient concentrations to cause a quick elimination of the target organisms might be unlikely [15].
The results shown in Table 6 presents the significantly prolonged MSP (P>0.05) of the infected mice, treated with the N. laevis and C. adansonii extracts compared to the group treated with normal saline. The combined extracts showed an MSP which was much lower than that for the positive control.
The P. berghei parasite could have developed resistance to chloroquine after its clear therapeutic effect and allowed the parasitemia to continue to be detectable in the blood of the mice treated with the standard drug [29]. The two plants we studied have been shown to possess anti-oxidants, anti-microbial and hepatoprotective properties [30]. These could be the reasons for the increased MSP of the mice despite their elevated parasitemia profiles.
A major pathologic characteristic of the mice infected with P. berghei is the loss of body weight [31]. In this context, our results as shown in Figures 4 and 5 are consistent with those found by Dikasso et al. [16], who reported reduced body weight in rodents infected with P. berghei and subsequently treated with the extract of Asparagus africanus. The loss of body weight might be dependent upon the availability of anti-nutritive factors in the crude extracts of both plants and the inhibitory effects of the combined extracts on food ingestion or the appetite [32].
Malaria infection is known to result in RBC destruction either by the parasite proliferation or by the action of spleen. The spleen is stimulated to produce many phagocytes upon the accumulation of abnormal RBCs in the blood [33]. In this study, the C. adansonii extract resulted in a lower PCV reduction than that caused by the N. laevis extract (Figures 6 and 7). It can be suggested that the C. adansonii contained trace minerals which are of immense benefit to the biosynthesis of the RBCs [3].
Limitation of the Study: The study was limited to the evaluation of the crude extracts of the leaves from the two plants. The fractionation, isolation and characterization of the bioactive agents from the plants were not performed. Future studies benefit from such characterizations.
Conclusions
The methanolic extract of N. laevis (200 mg/kg) showed a high inhibition of the parasite, while the ethylacetate extract of C. adansonii (600 mg/kg) produced the best inhibitory effect compared to that of the chlorquine treatment. However, the combination of the two extracts was not as effective as the individual ones. The results of this study validate the efficacy of the extracts of N. laevis and C. adansonii that are traditionally used in Nigeria for the treatment of the fever from malaria infection in human subjects.
Ethical Considerations
Compliance with ethical guidelines
We observed the principles regulating the use of experimental animals as outlined by the Committee on Ethics for Medical and Scientific Research at the Federal University of Technology, Minna, Nigeria. Also, the principles concerning the use and care of animals, as contained in the Canadian Council on Animal Care Guidelines and its review protocol, were complied fully in this study.
Funding
This research was part of an investigation on the Anti-plasmodial, Anti-inflammatory and Anti-nociceptive effects of leaf extracts of Newbouldia laevis and Crateva adansonii in rodents. This study is sponsored by the Tertiary Education Trust Fund (TETFUND) in Nigeria.
Author's contributions
All authors were instrumental in the successful completion of this study and contributed effectively to the preparation and revision of this article.
Conflict of interest
The authors declared no conflict of interest.
Acknowledgements
The authors appreciate the staff of the Department of Basic and Applied Sciences, Niger State Polytechnic P.M.B 01, Zungeru, Nigeria and the Biochemistry Department of the School of Life Sciences, Federal University of Technology, who contributed to this study. This study was funded by the authors. There was no conflict of interests whatsoever in conducting this study.
References
Haidet A. The medicinal value of the rainforest [Internet]. 2003 [Updated 2003 May 15]. Available from: http://jrscience.wcp.muohio.edu/fieldcourses03/PapersCostaRicaArticles/FinalPaper.TheMedicinalVa.html
Bloland PB, World Health Organization. Drug resistance in malaria [Internet]. 2001 [Updated 2001 April]. Available from: https://apps.who.int/iris/handle/10665/66847
Jigam AA, Akanya H, Dauda BEN, Ogbadoyi EO. Antiplasmodial, analgesic and anti-inflammatory effects of crude Guiera senegalensis Gmel (Combretaceae) leaf extracts in mice infected with Plasmodium berghei. J Pharmacogn Phytother. 2011; 3(10):150-4. [DOI:10.5897/JPP11.033]
White NJ, Olliaro PL. Strategies for the prevention of antimalarial drug resistance: Rationale for combination therapy for malaria. Parasitol Today. 1996; 12(10):399-401. [DOI:10.1016/0169-4758(96)10055-7]
Jigam AA, Akanya HO, Dauda BEN, Okogun JO. Polygalloyltannin isolated from the roots of Acacia nilotica Del. (Leguminoseae) is effective against Plasmodium berghei in mice. J Med Plants Res. 2010; 4(12):1169-75. https://academicjournals.org/JMPR/PDF/pdf2010/18June/Jigam%20et%20al.pdf
Basso LA, da Silva LHP, Fett-Neto AG, de Azevedo Junior WF, de Souza Moreira Í, Palma MS, et al. The use of biodiversity as the source of new chemical entities against defined molecular targets for treatment of malaria, tuberculosis, and T-cell mediated diseases - a Review. Mem Inst Oswaldo Cruz, Rio de Janeiro. 2005; 100(6):475-506. [DOI:10.1590/S0074-02762005000600001] [PMID]
Tu Y. Artemisinin—a gift from traditional Chinese medi-cine to the world (Nobel lecture), Angewandte Chemie (International Ed. in English), 2016; 55(35):10210-26. [DOI:10.1002/anie.201601967]
Usman H, Yaro AH, Garba MM. Analgesic and anti-inflammatory screening of Newbouldia laevis flower in rodents. Trends Med Res. 2008; 3(1):10-5. [DOI:10.3923/tmr.2008.10.15]
Adzu B, Haruna AK. Studies on the use of Zizyphus spina-christi against pain in rats and mice. Afr J Biotechnol. 2007; 6(11):1317-24. https://www.ajol.info/index.php/ajb/article/view/57480
Yaksh TL, Wallace MS. Opioids, analgesics and pain management. In: Brunton L, Chabner BA, Knollman B. Goodman and Gilman’s The Pharmacological Basis of Therapeutics. 12th ed. New York City: McGraw Hill Professional; 2011. p. 481-525. https://books.google.com/books?id=e_yAOpyyaowC&dq
Furst DE, Ulrich RW, Varkey-Altamirano C. Nonsteroidal anti-inflammatory drugs, disease-modifying antirheumatic drugs, nonopioid analgesics, & drugs used in gout. In: Katzung BG, Masters SB, Trevor AJ. Basic and Clinical Pharmacology. 11th ed. New York City: McGraw Hill Professional; 2009. 621-42. https://books.google.com/books?id=4O7ghcthkt4C&dq
Gamaniel KS. Toxicity from medicinal plants and their products. Niger J Nat Prod Med. 2000; 4:4-8. [DOI:10.4314/njnpm.v4i1.11729]
Tsado AN, Bashir L, Mohammed SS, Famous IO, Yahaya AM, Shu’aibu M, et al. Phytochemical composition and antimalarial activity of methanol leaf extract of Crateva adansonii in Plasmodium berghei infected mice. Br Biotechnol J. 2015; 6(4):165-73. [DOI:10.9734/BBJ/2015/16038]
Ukwuani-Kwaja AN, Adikwu JE, Abdullahi A. Acute oral toxicity and antipyretic activity of methanolic leaves extract of Newbouldia laevis and Securidica lungipedunculata in albino rats. Asian J Biochem Pharm Res. 2018; 8(1):67-72. [DOI:10.24214/AJBPR/8/1/6772]
Fidock DA, Rosenthal PJ, Croft SL, Brun R, Nwaka S. Antimalarial drug discovery: Efficacy models for compound screening. Supplementary documents. Trends Parasitol. 2004; 15:19-29. https://www.mmv.org/sites/default/files/uploads/docs/publications/8_-_supplementary_info_SCREENING_PDF_7.pdf
The Canadian Council on Animal Care. CCAC guidelines on: Animal use protocol review (1997) [Internet]. 1996 [Updated 1996 September 29]. Available from: https://norecopa.no/3r-guide/ccac-guidelines-on-animal-use-protocol-review
Lorke D. A new approach to practical acute toxicity testing. Arch Toxicol. 1983; 54:275-87. [DOI:10.1007/BF01234480] [PMID]
Dacie JV, Lewis SM. Practical haematology. 7th ed. New York: Churchill Livingstone; 1991. https://books.google.com/books?id=xHZrAAAAMAAJ&dq
Usman H, Osuji JC. Phytochemical and in vitro antimicrobial assay of the leaf extract of Newbouldia laevis. Afr J Tradit Complement Altern Med. 2007; 4(4):476-80. [DOI:10.4314/ajtcam.v4i4.31240]
Elujoba AA, Odeleye OM, Ogunyemi CM. Traditional medicine development for medical and dental primary health care delivery system in Africa. Afr J Tradit Complement Altern Med. 2005; 2(1):46-61. [DOI:10.4314/ajtcam.v2i1.31103]
Josephine Ozioma EO, Nwamaka Chinwe OA. Herbal medicines in African traditional medicine. In: Builders PF, editor. Herbal Medicine. London: IntechOpen; 2019. 191-214. [DOI:10.5772/intechopen.80348]
Ajaiyeoba E, Falade M, Ogbole O, Okpako L, Akinboye D. In vivo antimalarial and cytotoxic properties of Annona senegalensis extract. Afr J Tradit Complement Altern Med. 2006; 3(1):137-41. [DOI:10.4314/ajtcam.v3i1.31148]
Akanji MA, Salau AK, Yakubu MT. Safety evaluation of aqueous extract of Crateva adansonii leaves on selected tissues of rats. Fount J Nat Appl Sci. 2013; 2(1):17-28. https://www.academia.edu/download/42557647/Safety_Evaluation_of_Aqueous_Extract_of_20160210-3699-1v8pycu.pdf
Dikasso D, Mekonnen E, Debella A, Abebe D, Urga K, Makonnen W, et al. In vivo anti-malarial activity of hydroalcoholic extracts from Asparagus africanus Lam. in mice infected with Plasmodium berghei. Ethiop J Health Dev. 2006; 20(2):112-8. [DOI:10.4314/ejhd.v20i2.10021]
Muregi FW, Ishih A, Miyase T, Suzuki T, Kino H, Amano T, et al. Antimalarial activity of methanolic extracts from plants used in Kenyan ethnomedicine and their interactions with Chloroquine (CQ) against a CQ-tolerant rodent parasite, in mice. J Ethnopharmacol. 2007; 111(1):190-5. [DOI:10.1016/j.jep.2006.11.009] [PMID]
Carvalho LH, Brandão MG, Santos-Filho D, Lopes JL, Krettli AU. Antimalarial activity of crude extracts from Brazilian plants studied in vivo in Plasmodium berghei-infected mice and in vitro against Plasmodium falciparum in culture. Braz J Med Biol Res. 1991; 24(11):1113-23. [PMID]
Usha Devi C, Valecha N, Atul PK, Pillai CR. Antiplasmodial effect of three medicinal plants: A preliminary study. Curr Sci. 2001; 80(8):917-9. https://www.jstor.org/stable/24105798
Noedl H, Wongsrichanalai C, Wernsdorfer WH. Malaria drug-sensitivity testing: New assays, new perspectives. Trends Parasitol. 2003; 19(4):175-81. [DOI:10.1016/S1471-4922(03)00028-X]
Mengiste B, Makonnen E, Urga K. Invivo antimalarial activity of Dodonaea angustifolia seed extracts against Plasmodium berghei in mice model. Momona Ethiop J Sci. 2012; 4(1):47-63. [DOI:10.4314/mejs.v4i1.74056]
N’guessan JD, Coulibaly A, Ramanou AA, Okou OC, Djaman AJ, Guédé-Guina F. Antibacterial activity of Thonningia sanguinea against some multi-drug resistant strains of Salmonella enterica. Afr Health Sci. 2007; 7(3):155-8. [PMID] [PMCID]
Perlmann P Troye-Blomberg M. Malaria Immunology. 80th edition. Karger Pub, Stockholm; 2007. https://books.google.com/books?id=dSVWAQAAQBAJ&dq
Chinchilla M, Guerrero OM, Abarca G, Barrios M, Castro O. An in vivo model to study the anti-malaric capacity of plant extracts. Rev Biol Trop. 1998; 46(1):35-9. [PMID]
Taylor PJ, Hurd H. The influence of host haematocrit on the blood feeding success of Anopheles stephensi: Implications for enhanced malaria transmission. Parasitology. 2001; 122(5):491-6. [DOI:10.1017/S0031182001007776] [PMID]